Pulp biology
Headlines
External irritation may cause symptomatic or asymptomatic pulp inflammation
Inflammation may be spatially limited, but if the irritation is not removed, will lead to progressing necrosis
Degenerative changes occur even in healthy dental pulp tissue with age
Pulpal complications of caries, extensive wear or other external irritation may result in symptomatic or asymptomatic inflammation, followed by partial or progressive pulp tissue degradation and necrosis. Appropriate treatment of the diseased pulp aims to preserve the vitality of the pulp, either completely or partially, and can thus be regarded as “preventive endodontics”. Understanding of the physiology and pathology of the dentin-pulp complex is a prerequisite for proper diagnosis and treatment choice. This review describes the basic structure and physiology of the healthy dental pulp and the principles of the initiation and progression of inflammatory reactions in the low-compliance environment of the pulp chamber and root canals. The mechanisms of pain and hypersensitivity, as well as the means that the dentin-pulp complex may react to a repeated or persistent pain-producing irritation, are also discussed. The chosen treatment modalities of the vital pulp may vary from caries excavation and cavity sealing, partial or complete pulpotomy to pulpectomy, and will be discussed in detail in other articles in this issue dealing with the diagnostics, vital pulp therapies and emergency treatment.
Even though dentin is mineralized and the pulp a loose connective tissue, they form a developmentally interdependent and functionally integrated continuum, often referred to as the dentin‐pulp complex, where physiologic and pathologic reactions in one will also affect the other. Whereas dentin forms the main body of the tooth, providing support to enamel, resilience under occlusal loads, and physical protection of the pulp against microbes and other noxious substances, the pulp tissue readily reacts to the external irritation, initiating and orchestrating the defensive reactions that aim to keep the tooth functional and protect it from microbial invasion.
Dentin
Dentin is mineralized collagenous tissue, a nanocrystalline-reinforced collagen biocomposite, with 70 w-% (55 vol-%) biological hydroxyapatite (Ca10(PO4)6(OH)2) and 20 w-% (30 vol-%) organic components [1]. Major part of dentin is intertubular, formed by the dentin-forming odontoblasts at the dentin-pulp border. Tubular density in root dentin is lower than in coronal dentin, especially in the most apical part [2]. Peritubular (intratubular) dentin forms in a regular circular manner on the walls of the dentinal tubules.
Dentin-enamel junction (DEJ) provides the mechanical attachment of enamel to dentin. Together with the outermost mantle dentin with a gradual change of the mineralization rate towards the pulp, they create a 500 µm “resilience zone” necessary to prevent fractures under high occlusal forces [1].
Primary dentin formation (primary dentinogenesis) occurs during the formation and growth of the bulk of the crown and root, forming the main portion of dentin. Thereafter, dentin formation continues as secondary dentin at much slower rate throughout life, leading to gradual obliteration of the pulp chamber and root canals [1].
Tertiary dentin is formed as a response to external irritation, including wear and erosion, trauma, caries, cavity preparation and chemical irritation. The growth factors and other bioactive molecules present in mineralized dentin, liberated during caries or wear, are believed to initiate and control the tertiary dentin formation [3]. Tertiary dentin increases the mineralized barrier thickness between external irritation and pulp tissue, aiming to retain the pulp tissue vital and non-infected. There are two kinds of tertiary dentin, namely reactionary dentin, formed by original odontoblasts, and reparative dentin, formed by newly differentiated replacement odontoblasts [4]. Reactionary dentin is tubular and relatively similar to secondary dentin in structure, while reparative dentin (also called fibrodentin or even “calcified scar tissue” [4] is believed to be relatively impermeable, forming a barrier between tubular dentin and pulp tissue.
Pulp tissue and its homeostasis
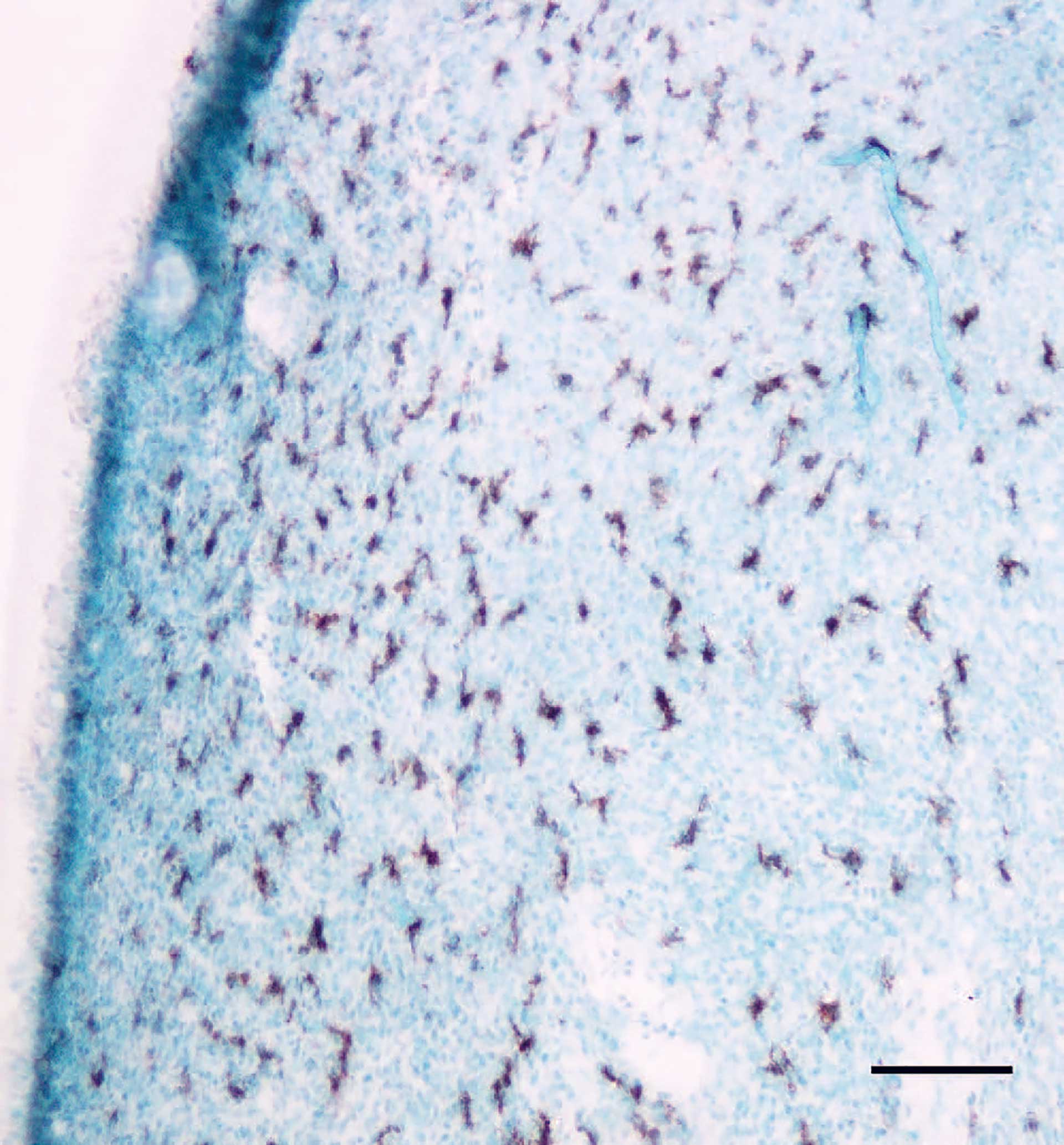
Figure 1. Macrophages in a mouse incisor identified with an antibody against LYVE-1. Bar: 100 µm.
Odontoblasts are the outermost cells of the pulp, forming a barrier between the pulp and dentin. This barrier may be disrupted as a response to trauma, dentin preparation or caries. Odontoblasts leave behind dentinal tubules, in which highly mineralized peritubular dentin is formed, leading to tubule occlusion that may be accelerated during external irritation [4].
The dental pulp tissue is a loose connective tissue well supplied by blood vessels and nerves. The connective tissue comprises the interstitial fluid and collagen-dominated matrix [5]. Most cells in the pulp are fibroblasts and undifferentiated cells (stem cells), which can differentiate into fibroblasts or replacement odontoblasts. Macrophages (figure 1), lymphocytes and sometimes some mast cells can also be seen. The presence of immune cells in the normal pulp indicates that the pulp is equipped to initiate immune responses.
Pulp tissue circulation and innervation
The highest density of vessels is in the most coronal part of the pulp. The arteries enter the pulp through the apex and leave the pulp as venules. A dense network of capillaries supplies the odontoblasts, as well as all other interstitial cells, with nutrients and oxygen.
The existence of lymphatic vessels in the pulp has been debated for many years, and such lymphatic vessels were previously thought to contribute to fluid volume control and drainage in the tissue [6]. More recent studies shows that the dental pulp lacks lymphatic vessels (7,8).
The pulp is equipped with a high number of axons, both myelinated and unmyelinated. The majority (approximately 90%) is sensory nerve fibers and the rest is of autonomic character. The myelinated nerve fibers are of sensory origin and separated in Aβand Að fibers. Under normal conditions they have a lower threshold than unmyelinated sensory C-fibers (table 1). The fibers are activated by different stimuli and evoke either “prepain” or pain perception.
Fiber type |
Diameter (µm) |
Conduction velocity (m/sec) |
Sensation |
Activation |
Terminal sites |
---|---|---|---|---|---|
A-β (7%) |
5-12 |
30-70 |
“Prepain”, sharp pain |
Pressure, touch, vibration |
Dentin, predentin, odontoblasts, pulp |
A-δ (93%) |
1-5 |
6-30 |
“Prepain”, sharp pain |
Heat, cold |
Dentin, predentin, odontoblasts, pulp |
C |
0,4-1 |
0,5-2 |
Ache |
Heat, infl. mediators |
Pulp, blood vessels |
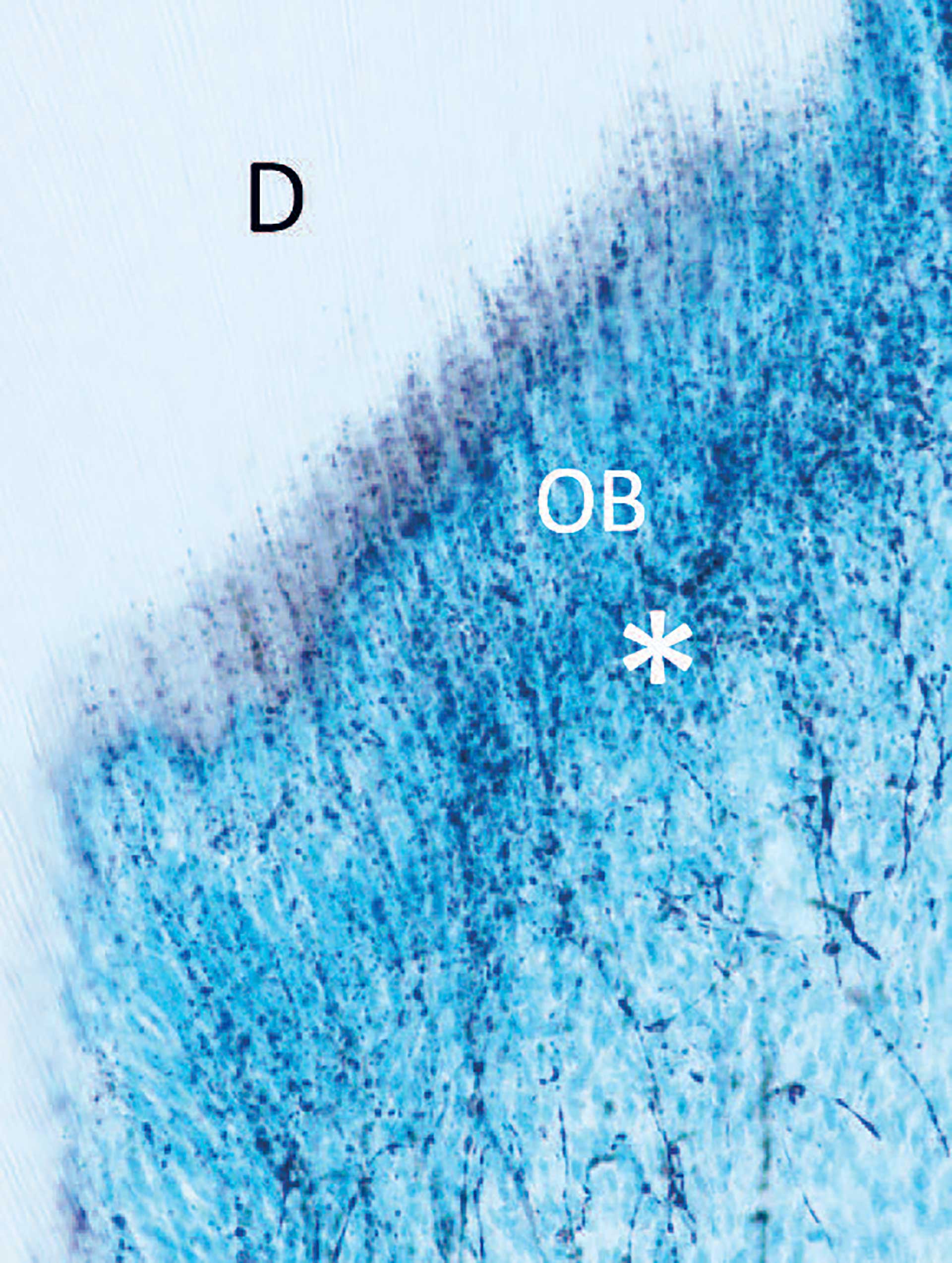
Figure 2. Section of a pulp horn from a rat molar, showing nerve fibers in the sub-odontoblastic region (*) with extentions crossing between the odontoblasts (OB), penetrating the inner part of dentin (D).
Both sensory and sympathetic nerve fibers accompany blood vessels and encircle them. While the sensory nerves emit a few branches in the radicular pulp, they branch extensively in the coronal pulp and form a subodontoblastic plexus. Some branches enter the dentinal tubules and reach the inner part of dentin (figure 2), especially under the cusps where also the tubule density is highest [9]. This may relate to the sensing of external irritation [10]. The nerve fibers are in intimate contact with odontoblasts and there may exist bidirectional communication [11].
Pulp inflammation
Vascular responses and fluid transport
Inflammation in the pulp takes place in a low compliant environment, between rigid dentinal walls. The acute vascular reactions to an inflammatory stimulus are vasodilatation and increase in interstitial fluid volume due to increased vascular permeability, leading to a relatively large increase in the hydrostatic pressure in the pulp [12]. If the inflammatory response is spatially limited, fluid absorption occurs into nearby blood vessels [12] or by transportation toward non-inflamed lower pressure areas probably in loose connective tissue gaps toward apex [6] (figure 3). The drainage capacity can explain why an elevated tissue pressure in inflamed pulps may persist locally for a long time [13] without extensive damage to the pulp. If the inflammatory agents are removed, a normalization of pressure can take place and healing of the tissue occur (reversible pulpitis). However, a significant increase in interstitial fluid pressure, due to a strong and widespread inflammatory response, may seriously impede pulpal blood flow by compression of venules which contribute to pulp necrosis development. Other contributing factors to necrosis development can take place when the endothelium is exposed to bacterial endotoxins. The endothelium will respond with production of vasoconstrictors that will reduce blood flow [14] and is also shown to participate in procoagulant reactions that promote fibrin clot formation [15].
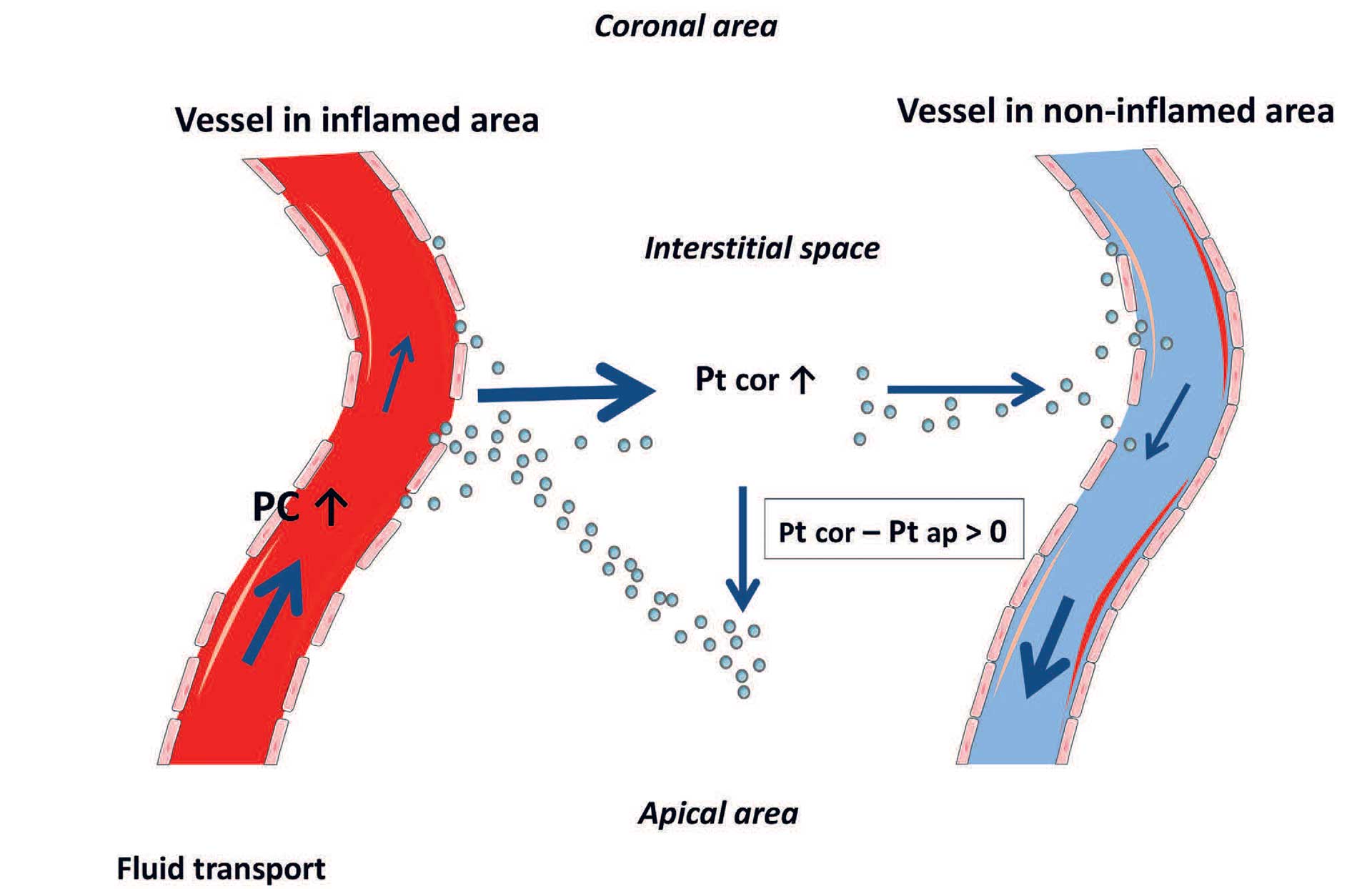
Figure 3. Fluid and plasma protein (dots) removal from inflamed (left) and un-inflamed (right) coronal pulp tissue. Arrows indicate relative magnitude and direction of fluid transport (Pc, capillary blood pressure; Pt, tissue pressure; ) in interstitial fluid. cor = coronal, ap = apical. Reprinted with permission from [6].
Immune cells in pulp
Resident immune cells in the pulp can respond to an inflammatory or infectious insults by innate and adaptive immune responses [16] [17]. Inflammatory mediators initiating these responses can be released from carious dentin, odontoblasts, or resident pulp cells.
Odontoblasts represent the first line of detection and defense against external irritants and participate in the initiation of inflammatory and/or immune responses. They express Toll‐like receptors (TLRs), a group of transmembrane glycoproteins, that recognize various elements of microbes, viruses, and fungi [18] [19]. After early activation of the odontoblasts, innate immune responses are initiated by recruitment of immune cells, production of antimicrobial peptides, and maturation of dendritic cells [18]. They secrete several products with a broad spectrum of antimicrobial and immunomodulatory activities [20] [21], such as the induction of pro-inflammatory cytokine production in immune cells, chemoattraction, dendritic cell maturation and macrophage differentiation. Proteinase‐activated receptors (PARs) are receptors that undergo proteolytic activation by proteases. They participate in controlling a wide range of biological processes, such as inflammation, hemostasis, thrombosis, and hard tissue formation. PAR‐1 and ‐2 are present in human odontoblasts [22]. The expression is significantly increased in response to caries, both in the odontoblasts [22] and in pulp tissue, indicating a regulatory role in reparative dentin formation and/or in pulp inflammation.
Resolution or advancement of the initial inflammation is further regulated by a complex network of inflammatory chemokines which promote recruitment of immune cells and regulate other inflammatory responses. The dendritic cells migrate to regional lymph nodes for antigen-presentation to naïve T-cells. Activated T cells can then enhance B-cell proliferation, followed by development of antibody-producing plasma cell. Neutrophils and macrophages are professional phagocytes in innate immune responses and are recruited to the pulp in the initial phase of inflammation.
Dentin-pulp nociception and hypersensitivity
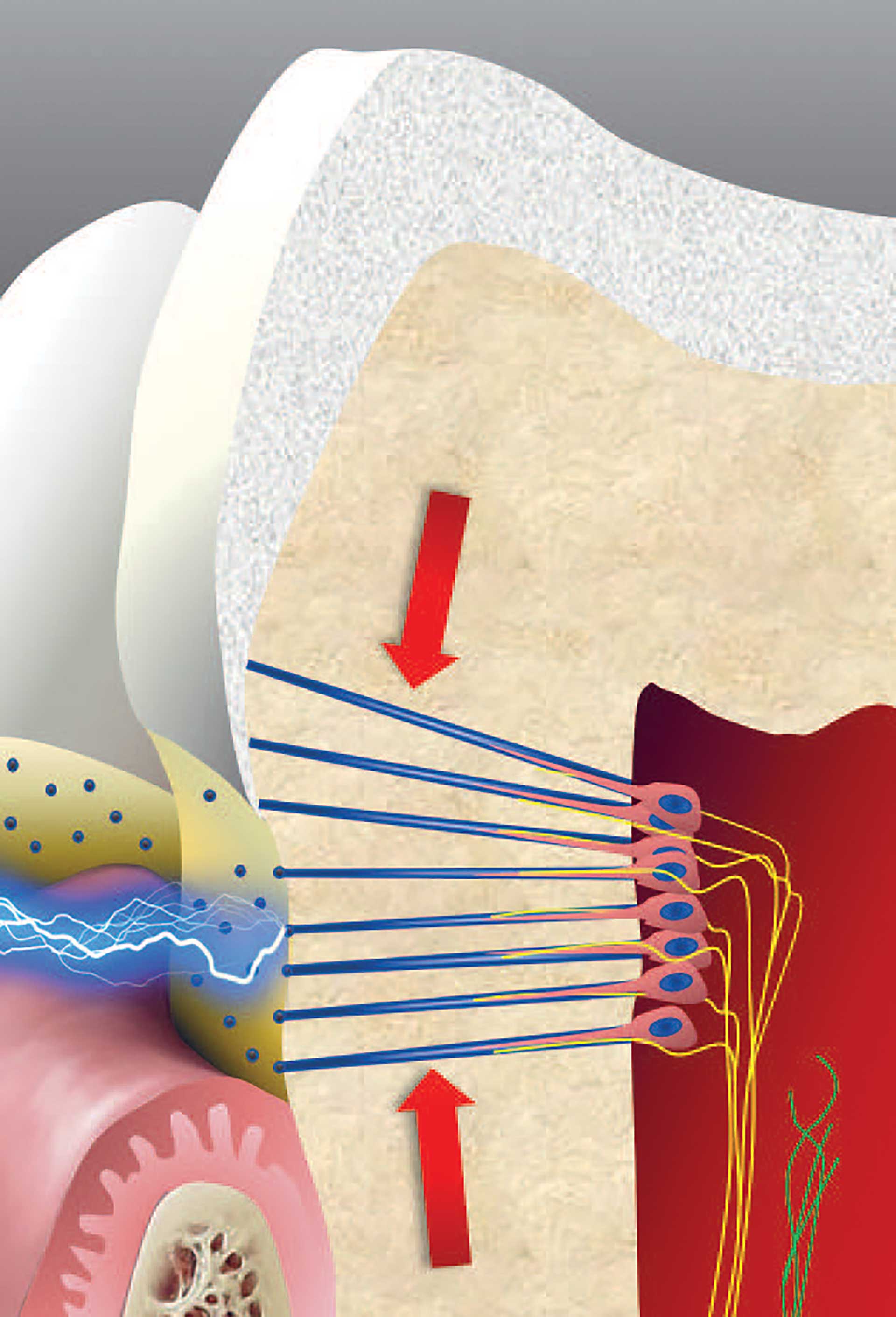
Figure 4. Intradental myelinated A-fibers (yellow) have their nerve terminals situated in the pulp periphery, crossing between odontoblasts, and innervating the inner part of dentin. A-fibers have a relatively low threshold and give a sharp and pricking sensation. They can be activated by mechanical compression forces (arrows) and external stimuli like cold and hot causing fluid flow. Dentinal tubules (green) may be exposed in areas not covered by cementum or enamel. Unmyelinated C-fibers have normally a relatively high threshold that is lowered following tissue injury and inflammation. C-fibers give rise to the unpleasant burning and acing sensation often experienced following tissue injury.
The space between the odontoblast process and tubule wall is filled with dentinal fluid, and the movement of this fluid is considered a main factor involved in dentinal pain transmission. Pain-producing stimuli, including heat and cold, air blasts and probing on denuded dentin, can displace the tubular fluid [23]. This is referred to as the hydrodynamic mechanism of dentin sensitivity, involving both fluid movement and mechanotransduction. Classical mechanoreceptors have been demonstrated on pulpal afferent nerves innervating the inner part of dentin [24] (figure 4) and in the odontoblasts [25] [26] [27] [28], thus providing a mechanistic support to the theory. Dentinal fluid movement is translated into electric signals by activation of mechanosensitive ion channels [29].
It is mainly the A-fibers (Table 1), located in the inner part of dentin, that are activated during displacement of the tubular contents [30] (figure 4). In other tissues, e.g. skin and gingiva, A-fibers transmit un-painful stimuli like touch and tactile information. Most neurons innervating teeth have the same A-fiber characteristics typical for low threshold mechanoreceptors. The different sensation of the mechanosensitive A-fibers in teeth may be due to their arrangement with projections to pain sensing neurons located more centrally [31]. The polymodal C-fibers, on the other hand, are the classical nociceptive fibers. Their nerve terminals are located deeper in the pulp tissue and are normally silent in the uninflamed pulp. The C-fibers have numerous receptors that can be activated during inflammation by heat and other types of stimuli [32]. Particularly, a receptor termed the “transient receptor potential, subtype vanilloid 1” or TRPV1, responds to heat, inflammatory mediators and acidity (pH <6) [33]. Along with the inhibition of Ca+ and K+ channels in dental afferent neurons [34] [35] [36], eugenol desensitizes TRPV1 [37], an action that may be involved in the anodyne action of zinc oxide eugenol.
Inflammation and hypersensitivity
Hypersensitivity of dentin may also be explained by the hydrodynamic theory. In addition to sprouting of nerve fibers during inflammation (figure 5), new sodium channels, capable of activating nerves, are upregulated in the nerve fibers under inflammatory conditions [38]. An increase in the density of both nerves and sodium channels increases the sensitivity of dentine, thus resulting in hypersensitivity. Hypersensitivity also typically occurs in the cervical area where the dentin is exposed because the protective enamel/cement is worn out or etched away (figure 4). The odontoblasts and/or pulp cells respond by forming intratubular deposits or eventually tertiary dentin. This results in narrowing or closing of the dentinal tubules. Deposition of tertiary dentin leads to decreased conductivity compared to the primary and secondary dentin. This may also reduce the sensitivity, as reparative dentin is less innervated by sensory nerve fibers.
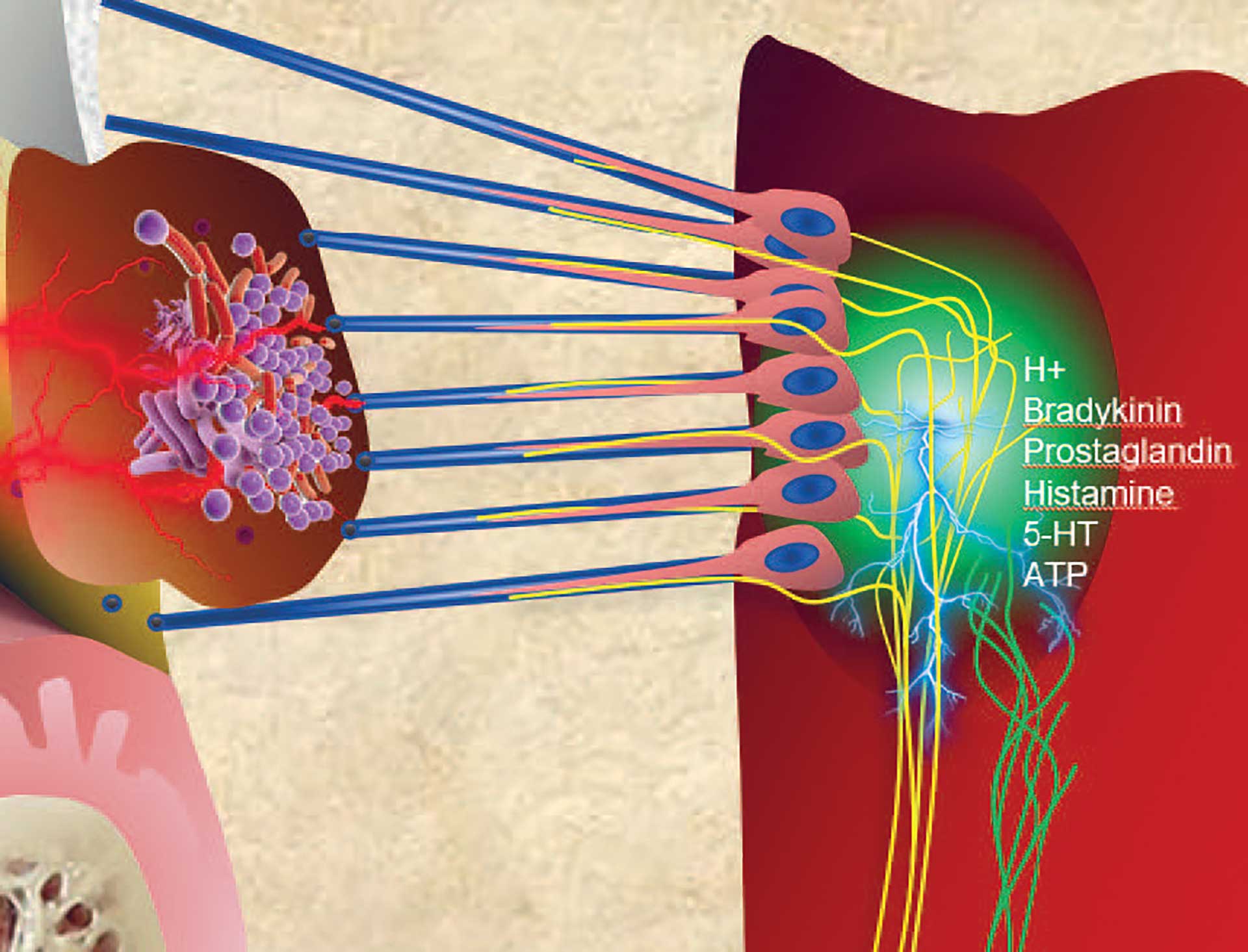
Figure 5. During inflammatory conditions, as shown under a carious lesion, the nerve fibers have an ability to branch and extend their receptive fields. Combined with a change in receptor expression on their surface, the nerves become more sensitive to external stimuli.
Age changes in the dentin-pulp complex
Structural changes
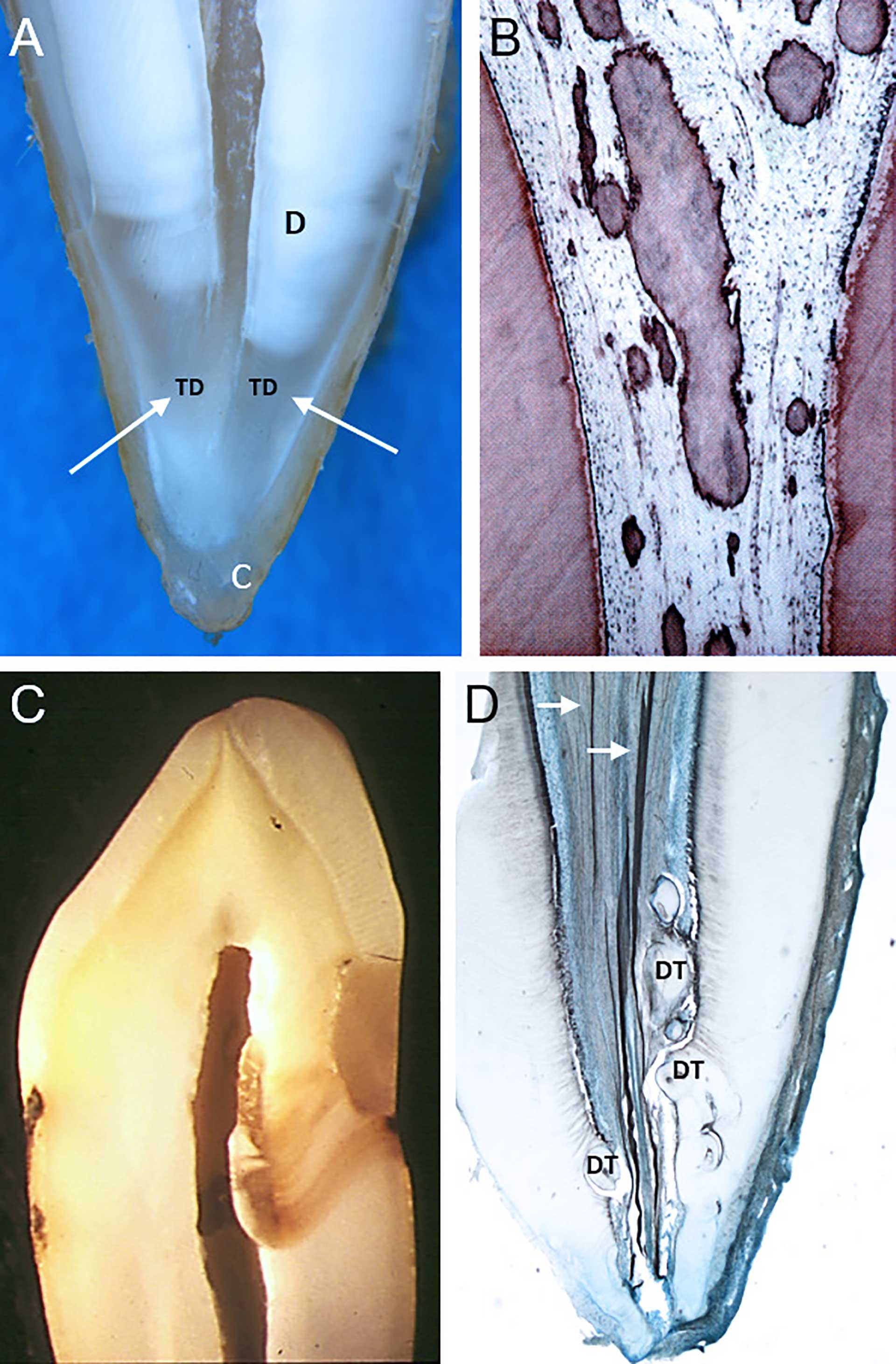
Figure 6. Age changes in the pulp. (A) Translucent dentin formation (TD and white arrows), starting in the apical area and proceeding coronally, causes a gradual closure of the dentinal tubules, rendering them impermeable to bacterial colonization. Also continued cementum formation (C) causes a change in the anatomical landmarks. (B) Dystrophic or diffuse calcifications in an aged pulp. (C) “Scar tissue” in the pulp, presented by tertiary dentin under a restauration with marginal leakage. Extensive cavity preparations, resulting in large amounts of tertiary dentin, may compromise the pulpal circulation. (D) Denticles (DT), both embedded and free, are relative common in the pulp tissue. They may increase in size during aging. White arrows points at nerve fibers in the root. Figure B and C reprinted with permission from the Norwegian Dental Journal [46].
Aging results in cellular and extracellular changes of the dentin-pulp complex. Formation of the highly mineralized peritubular dentin causes a reduction in tubular lumen diameter, even with complete occlusion of the tubule. Age-related root tubular sclerosis starts from the apical region (figure 6A) and advances coronally [39] [40], influencing root dentin permeability [41] [42]. This may thus reduce bacterial penetration of dentinal tubules in elderly. Aging odontoblasts show a reduction in cell organelles, together with reduced size and polarization [43]. Pulp cell density is reduced, and the number of nerves and blood vessels is decreased [44]. The amount of collagen in the pulp increases, resulting in fibrotic changes, whereas calcifications, both diffuse and localized, are more common (figure 6B and C). Although pulp stones are considered as normal anatomical entities (figure 6D), their size may increase during aging. Diffuse mineralization, however, is a result of age changes or inflammatory conditions in the dental pulp [45].
Dimensional changes
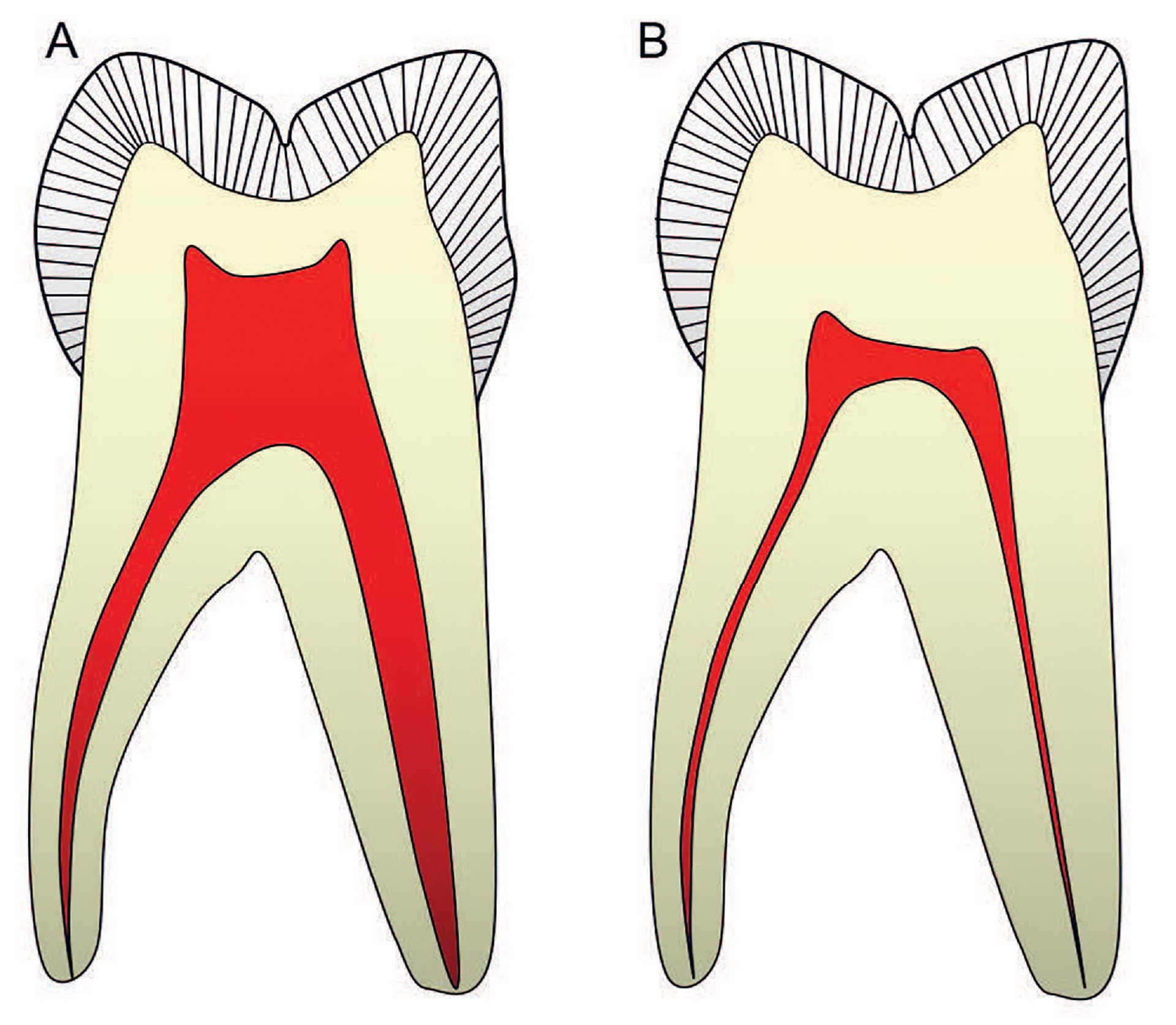
Figure 7. The most common changes in a molar tooth will be flattening of the pulp chamber due to dentin production in the pulp roof and floor, combined with narrowing of the root canals. Reprinted with permission from the Norwegian Dental Journal [46].
An aged pulp characterized by “restorative scar tissues”, fibrosis and mineralization will have a reduced draining capacity, thus making it more vulnerable to circulatory failure [6]. To counteract these structural changes, increased dentin thickness may protect the pulp from external stimuli. Over time there will be a progressive linear reduction in the pulp volume, due to the continued production of dentin [46]. This process can be accelerated due to irritation from caries, restorations, or periodontal disease (figure 7). Also, cementum apposition over years may change the anatomical landmarks in the apical region (figure 6A).
References
Tjäderhane L, Carrilho MR, Breschi L, Tay FR, Pashley DH. Dentin basic structure and composition - an overview. Endod Topics. 2012; 20:3-29.
Mjør IA, Smith MR, Ferrari M, Mannocci F. The structure of dentine in the apical region of human teeth. Int Endod J. 2001; 34:346-53.
Smith AJ, Scheven BA, Takahashi Y, Ferracane JL, Shelton RM, Cooper PR. Dentine as a bioactive extracellular matrix. Arch Oral Biol. 2012; 57:109-21.
Tjäderhane L, Haapasalo M. The dentin–pulp border: a dynamic interface between hard and soft tissues. Endod Topics. 2012; 20:52-84.
van Amerongen JP, Lemmens IG, Tonino GJ. The concentration, extractability and characterization of collagen in human dental pulp. Arch Oral Biol. 1983; 28:339-45.
Berggreen E, Wiig H, Virtej A. Fluid transport from the dental pulp revisited. Eur J Oral Sci. 2020; 128:365-8.
Gerli R, Secciani I, Sozio F, Rossi A, Weber E, Lorenzini G. Absence of lymphatic vessels in human dental pulp: a morphological study. Eur J Oral Sci. 2010; 118:110-7.
Lohrberg M, Wilting J. The lymphatic vascular system of the mouse head. Cell Tissue Res. 2016; 366:667-77.
Byers MR, Suzuki H, Maed T. Dental neuroplasticity, neuro-pulpal interactions, and nerve regeneration. Microsc Res Tech. 2003; 60:503-15.
Tjäderhane L, Paju S. Dentin-pulp and periodontal anatomy and physiology. In: Ørstavik D, editor. Essential Endodontology: Prevention and Treatment of Apical Periodontitis. 3rd edition. Hoboken: John Wiley & Sons Ltd; 2020. p. 11-58.
Kido MA, Ibuki T, Danjo A, Kondo T, Zhang JQ, Yamaza T & al. Immunocytochemical localization of the neurokinin 1 receptor in rat dental pulp. Arch Histol Cytol. 2005; 68:259-65.
Heyeraas KJ, Berggreen E. Interstitial fluid pressure in normal and inflamed pulp. Crit Rev Oral Biol Med. 1999; 10:328-36.
Tønder KJ, Kvinnsland I. Micropuncture measurements of interstitial fluid pressure in normal and inflamed dental pulp in cats. J Endod. 1983; 9:105-9.
Okiji T, Morita I, Sunada I, Murota S. Involvement of arachidonic acid metabolites in increases in vascular permeability in experimental dental pulpal inflammation in the rat. Arch Oral Biol. 1989; 34:523-8.
Stern DM, Bank I, Nawroth PP, Cassimeris J, Kisiel W, Fenton JW 2nd & al. Self-regulation of procoagulant events on the endothelial cell surface. J Exp Med. 1985; 162:1223-35.
Hahn CL, Liewehr FR. Innate immune responses of the dental pulp to caries. J Endod 2007; 33:643-51.
Hahn CL, Liewehr FR. Update on the adaptive immune responses of the dental pulp. J Endod. 2007; 33:773-81.
Farges JC Keller JF, Carrouel F, Durand SH, Romeas A, Bleicher F & al. Odontoblasts in the dental pulp immune response. J Exp Zool B Mol Dev Evol. 2009; 312b:425-36.
Pääkkönen V, Rusanen P, Hagström J, Tjäderhane L. Mature human odontoblasts express virus-recognizing toll-like receptors. Int Endod J. 2014; 47:934-41.
Mookherjee N, Brown KL, Bowdish DM, Doria S, Falsafi R, Hokamp K & al. Modulation of the TLR-mediated inflammatory response by the endogenous human host defense peptide LL-37. J Immunol. 2006; 176:2455-64.
Semple F, Dorin JR β-Defensins: Multifunctional modulators of infection, inflammation and more? J Innate Immun. 2012; 4:337-48.
Alvarez MMP, Moura GE, Machado MFM, Viana GM, de Souza Costa CA, Tjäderhane L & al. PAR‐1 and PAR‐2 expression is enhanced in inflamed odontoblast cells. J Dent Res. 2017; 96:1518-25.
Matthews B, Vongsavan N. Interactions between neural and hydrodynamic mechanisms in dentine and pulp. Arch Oral Biol. 1994; 39(Suppl):87S-95S.
Hermanstyne TO, Markowitz K, Fan L, Gold MS. Mechanotransducers in rat pulpal afferents. J Dent Res. 2008; 87:834-8.
Magloire H, Lesage F, Couble ML, Lazdunski M, Bleicher F. Expression and localization of TREK-1 K+ channels in human odontoblasts. J Dent Res. 2003; 82:542-5.
Magloire H, Couble ML, Thivichon-Prince B, Maurin JC, Bleicher F. Odontoblast: a mechano-sensory cell. J Exp Zool B Mol Dev Evol. 2009; 312b:416-24.
Magloire H, Maurin JC, Couble ML, Shibukawa Y, Tsumura M, Thivichon-Prince B & al. Topical review. Dental pain and odontoblasts: facts and hypotheses. J Orofac Pain. 2010; 24:335-49.
Chung G, Jung SJ, Oh SB. Cellular and molecular mechanisms of dental nociception. J Dent Res. 2013; 92:948-55.
Vongsavan N, Matthews B. The relationship between the discharge of intradental nerves and the rate of fluid flow through dentine in the cat. Arch Oral Biol. 2007; 52:640-7.
Närhi M. Activation of dental pulp nerves of the cat and the dog with hydrostatic pressure. Proc Finn Dent Soc. 1978; 74(Suppl 5-7):1-63.
Marfurt CF, Turner DF. The central projections of tooth pulp afferent neurons in the rat as determined by the transganglionic transport of horseradish peroxidase. J Comp Neurol. 1984; 223:535-47.
Närhi M, Yamamoto H, Ngassapa D. Function of intradental nociceptors in normal and inflamed teeth. In: Shimono M, Maeda T, Suda H, Takahashi K, editors. Dentin/pulp complex. Tokyo: Quintessence Publishing Co; 1996. p. 136-40.
Chaudhary P, Martenson ME, Baumann TK. Vanilloid receptor expression and capsaicin excitation of rat dental primary afferent neurons. J Dent Res. 2001; 80:1518-23.
Lee MH, Yeon KY, Park CK, Li HY, Fang Z, Kim MS & al. Eugenol inhibits calcium currents in dental afferent neurons. J Dent Res. 2005; 84:848-51.
Li HY, Park CK, Jung SJ, Choi SY, Lee SJ, Park K & al. Eugenol inhibits K+ currents in trigeminal ganglion neurons. J Dent Res. 2007; 86:898-902.
Chung G, Rhee JN, Jung SJ, Kim JS, Oh SB. Modulation of CaV2.3 calcium channel currents by eugenol. J Dent Res. 2008; 87:137-41.
Park CK, Kim K, Jung SJ, Kim MJ, Ahn DK, Hong SD & al. Molecular mechanism for local anesthetic action of eugenol in the rat trigeminal system. Pain. 2009; 144:84-94.
Fristad I, Närhi M. Dentinal and pulpal pain. In: Bjørndal L, Kirkevang LL, Whitworth J, editors. Textbook of Endodontology. 3rd ed. London: Wiley Blackwell; 2018. p. 33-48.
Nalbandian J, Gonzales F, Sognnaes RF. Sclerotic age changes in root dentin of human teeth as observed by optical, electron, and x-ray microscopy. J Dent Res. 1960; 39:598-607.
Vasiliadis L, Darling AI, Levers BG. The amount and distribution of sclerotic human root dentine. Arch Oral Biol. 1983; 28:645-9.
Paque F, Luder HU, Sener B, Zehnder M. Tubular sclerosis rather than the smear layer impedes dye penetration into the dentine of endodontically instrumented root canals. Int Endod J. 2006; 39:18-25.
Thaler A, Ebert J, Petschelt A, Pelka M. Influence of tooth age and root section on root dentine dye penetration. Int Endod J. 2008; 41:1115-22.
Couve E. Ultrastructural changes during the life cycle of human odontoblasts. Arch Oral Biol. 1986:31:643–51.
Bernick S, Nedelman C. Effect of aging on the human pulp. J Endod. 1975; 1:88-94.
Sayegh FS, Reed AJ. Calcification in the dental pulp. Oral Surg Oral Med Oral Pathol. 1968; 25:873-82.
Kristoffersen Ø, Nag OH, Drageset J, Fristad I. Endodontisk behandling hos eldre. Diagnostiske og behandlingstekniske utfordringer. Nor Tannlegeforen Tid. 2012; 122:510-5.
Corresponding author: Leo Tjäderhane, Department of Oral and Maxillofacial Diseases, University of Helsinki, PO Box 41, 00014 University of Helsinki, FINLAND. E-mail: leo.tjaderhane@helsinki.fi
Accepted for publication 01.08.2022
The article is peer reviewed.
Tjäderhane L, Berggreen E, Fristad I. Pulp Biology. Nor Tannlegeforen Tid. 2023; 133: 10-6.
Key words: dentin; dental pulp; inflammation; hypersensitivity