The handling of digital images
Authors
Digital imaging has revolutionized radiology during the past 20 years. All digital imaging is still based on analogue signals from imaging detectors which are converted into digital binary format which can be processed by computers. Digital images are formed of individual pixels, each containing contrast value (gray scale). Picture archiving and communication systems (PACS) provide tools for storing and transporting the digital images. The DICOM provide the universal format for the medical image data. Also other formats, e.g. bitmap (bmp) and jpeg are used for dental images. Digital imaging can be described in three steps: 1) image acquisition uses physical processes to get the analogue signal which is converted into digital raw data, 2) post-processing enables modification of the raw data to emphasize the diagnostic target features, 3) image review brings the processed images to the medical display. Quality assurance must cover the whole imaging chain. Due to the complex bony anatomy of the maxillofacial region, the 3D cone-beam computed tomography has become more popular in dental use. In the future, multimodal and 3D/4D imaging methods produce increasing data loads in radiology. Information concerning efficacy and effectiveness will be the fundamental motivator of the future radiological applications and IT solutions.
Digital imaging and IT has revolutionized radiology during the past 20 years, including data storage, transmission, image post-processing, analysis and review. Steps of digital imaging for optimization: image acquisition, post-processing, image review. Quality assurance must cover the whole imaging chain. CBCT 3D-imaging has become popular in dental radiology due to the complex bony anatomy of the maxillofacial region.
Advances in information technology have further expanded the scope of digital imaging modalities that have large impact also on the dentistry practice (1, 2). All digital imaging is still based on analogue signals from imaging detectors which are converted into digital binary format which can be processed by computers (3).
Digital images are formed of individual pixels. Each pixel in the image contain certain contrast value which tells how dark or bright (gray scale) the pixel will look in the image (see figure 1). Pixels are located as matrix in the image. The number of rows and columns determine the size of the image. The bit depth tells the dynamic range of the pixel - i.e. how many contrast values can be assigned to an individual pixel. Typically the bit depth can be in the order of 8 - 12 bits which means a gray scale range of 256 to 4096 values (as e.g. 8 bits provide 28 = 256 possible values). The image should typically be reviewed in 1: 1 (100 %) size which means that individual pixels will not be shown separately to the reviewer. The image contrast and brightness settings provide a possibility to accentuate a certain diagnostically relevant sub-sector of gray scales, depending of the examination (see figure 2). In radiology, this is referred to as windowing. However, this option is limited in dental practice.
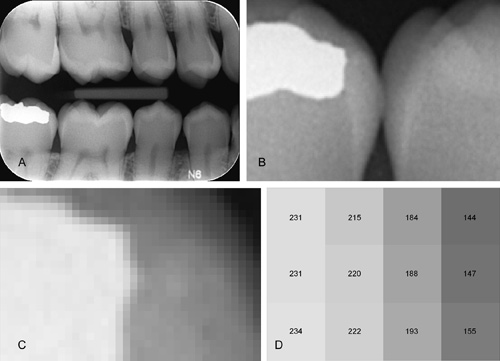
Figure 1. An example of digital image data (bitewing). The image contains rows and columns of individual pixels, each having their own gray scale pixel value. The original image (A), zoomed portions of the image (B-D) where pixel values corresponding to gray scale values have been marked (D). In x-ray images, the gray scale value of the pixel depends on the amount of radiation incident to the detector at the pixel location.
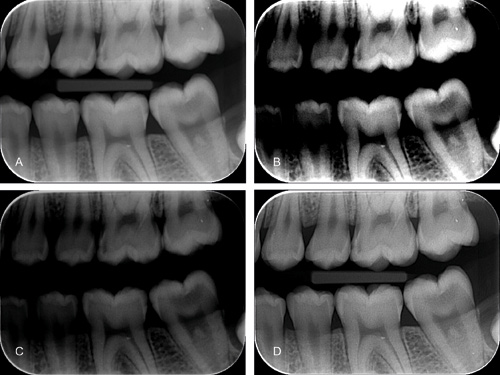
Figure 2. Example of the image post-processing to bitewing picture (A) including contrast (B) and brightness (C) adjustments in addition to edge-enhancement (D).
In terms of practicality, digital imaging has given unparallel possibility for image communication and access. In addition to image archiving and communication aspects, image post-processing enables enhancement of diagnostically important features from the wide dynamic range of pixel values in the digital data, well beyond the dynamic range of human visual system.
PACS and image format
Picture archiving and communication systems (PACS) were motivated by the development of digital imaging. PACS systems gradually displaced traditional film based image distribution in the early 2000. The idea was that if the potential of digital imaging was fully utilized in radiology, there must also be an efficient and universal method of storing and transporting the digital information. However, before that could be realized, manufacturers had to come into consensus on the common interface between the imaging equipment and the PACS networks. In the mid 80's, the American College of Radiology (ACR) and National Electrical Manufacturers Association (NEMA) developed a standard known as Digital Imaging and Communication in Medicine (DICOM). The DICOM provided the universal format for the medical image data and in combination with the Ethernet TCP/IP protocol, the image data could be managed from the imaging equipment to the PACS and the image display (3, 4, 5). The DICOM image contains the image data but also metadata describing identification and modality settings, etc. The contrast information in the DICOM image data has 16 bits as the dynamic range (16 bits can provide 216 = 65 536 gray scales). This contrast scale can be mapped for modality and clinical target based look-up tables which mean that the final image appearance at the image display can be automatically adjusted for optimal diagnostic review. The DICOM standard has been adopted by the majority of medical products also outside the radiology.
Digital images can be saved in various formats depending on dental x-ray equipment vendor. Bitmap image (bmp) is a traditional example of non-compressed lossless format where the image data contains fully the original pixel information. This also means that the file size in bitmap images is typically large, depending of the dental image resolution (pixel matrix size of the image). Compressed image formats reduce file size, utilizing either lossless compression - where original image data can be restored completely - or lossy compression, where the reduction in file size can be higher but the original pixel values cannot be fully recovered. Jpeg is mostly used format for compressed images. As the compression level in jpeg images is adjusted higher, the file size decreases but the data also becomes more «lossy» which means that the original pixel values cannot be fully recovered. Thus, the compression level should be selected appropriately for medical images. In radiological environment, the DICOM is - and should be - the predominant format for medical images (3).
The file size also affects to the image transmission time from a location to another. With a modern broadband network connection and for a single dental image, the transmission time is short (a few seconds) and it should not have any effect on clinical workflow. However, for multiple images or 3D datasets that may easily contain 100 MB of data, the transmission time can increase substantially and this should be taken into account when accessing data in remote locations.
Digital imaging process and quality assurance
Digital imaging can be described in three steps. In the first phase - image acquisition - the physical processes (e.g. x-ray attenuated through the patient) are utilized to get the analogue signal which is converted into digital raw data. In the second phase - post-processing - the raw data is modified through examination based algorithms in order to emphasize the diagnostic target features of the image data. In the last part - image review - the processed image data is brought to the medical display for radiologist or clinician interpretation (3).
Important aspect for the overall quality and radiological process flow is that all these three steps can be performed and optimized independent of each other. Thus, imaging devices and imaging parameters are updated as the technology allows more efficient detectors for raw data acquisition. Updating image processing software and different post-processing settings can highlight different anatomical targets from the same raw data. For example, different image reconstruction algorithms or gray scale window of the CT data can show either soft tissue contrast scale or high detail bone structures, depending of the clinical interest.
Despite of the independent parts in the digital imaging chain, the chain itself should be optimized as a holistic view. This also holds for quality assurance. The quality assurance protocol in digital radiology must be designed to include all phases from image data acquisition and scan parameter consistency to the image review. The quality chain is as strong as its weakest link - thus, even the preceding image acquisition and processing parts were well managed, if the image displays are not performing properly or the images are reviewed in sub-optimal ambient lighting, the diagnostic accuracy could be compromised (6, 7). Ambient lighting should be in the range of 15 - 20 lux for radiological image review, which is well below typical dental office lighting level (about 800 - 1000 lux). However, reduced lighting level cannot be performed during clinical work - thus, acquired images should be reviewed second time after the day in optimal ambient lighting conditions (8).
Evolution of dental imaging from 2D to 3D
Traditional image data in dental radiology has been mainly projected x-ray images, either intraoral, cephalometric or panoramic surveys (2). The digital image detectors include either storage phosphor based computed radiography systems (CR) or direct radiography (DR) systems with flat panel detectors. The flat panel detectors are steadily surpassing CR technology due to improved overall efficiency and image quality. Still, the projection images of the maxillofacial are include overlapping anatomy and geometrical variations which in many cases render them suboptimal for accurate linear measurements and superimposing bone structure evaluations.
The complex bony anatomy of the maxillofacial region has motivated more comprehensive and geometrically robust imaging methods which call for three-dimensional (3D) tomographic image data. With 3D data given by scanners such as multi-slice CT (MSCT) or dedicated dental cone-beam CTs (CBCT), versatile sectional views can be provided for dentists and dental radiologists (see figure 3) (9, 10). CT scan involves x-ray tube and detector rotation around the patient, to acquire sufficient projection samples from different directions. Those projections are then used as the raw data for tomographic image section reconstruction performed by the scanner computer (3). As the result, volumetric 3D image data is produced with much more extensive diagnostic value than mere projection image. However, as the computed tomography is also related with higher radiation exposure to the patient compared with panoramic or intraoral imaging, the indications for dental 3D CT imaging are confined mainly to pre-operative planning and post-operative evaluations. Dental CBCT scanners offer light-weight and more economical solution compared to MSCT - and typically also with higher spatial resolution and lower radiation exposure (11) - which has made them an attractive choice for dental «in-office» practices with limited space. If soft tissue characterisation is sought, magnetic resonance imaging (MRI) will provide superior contrast compared to x-ray methods (CBCT) and without exposure to ionizing radiation. However, MRI is more expensive radiological examination and hardly accessible for general dental practice.
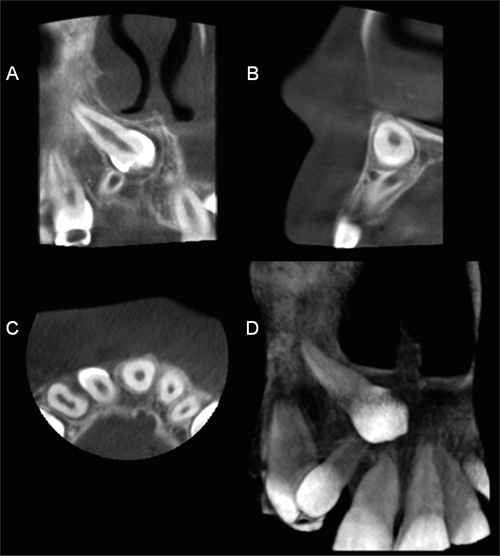
Figure 3. Cone-beam computed tomography (CBCT) image of the unerupted canine (D.13) including coronal (A), sagittal (B), axial (C) and 3D volume rendered view (D). CBCT imaging offers three-dimensional image data of the anatomy. Visualized image cross-sections can be oriented to conform any desired slice of the anatomy. The versatility of computed tomography is highly valuable for accurate imaging of maxillofacial structures which include complex 3D bone structures.
Digital dental photography is also part of the patient imaging where digital photos are used in the documentation of examination, treatment planning, monitoring and also the in evaluation of treatment outcome (12, 13). The digital photos are usually archived in the same database with the radiological images and reviewed with similar software. On the contrary to radiography, there are no harmful biological effects in photography, which is an important aspect when children are concerned. The benefits of photos are widely recognized for example in orthodontics, where they are used in analyzing the facial profiles. Dental photographs are also beneficial in monitoring soft tissue or mucous membrane lesions. Communication with patients and dental technicians is improved when dental photos are available. Patients seldom have sufficient knowledge of dental and periodontal diseases and photos can be used in patient education and motivation since pictorial presentation is often an effective method compared to verbal education. Dental technicians have better prospects to meet patients' and dentists' aesthetic demands in prosthetic restorations when photos are available. However, this may be challenging from the data privacy aspect if the technician works in another organization.
Future of radiological IT systems
Currently, PACS solutions have grown from early applications of data storage and transmission to modern radiological process control systems, with speech recognition based reporting (14, 15) and integration to the radiological information systems (RIS). The connection between the PACS and the RIS is essential since both systems direct the diagnostic process flow where bottlenecks can cause delays in a busy practice. The RIS and hospital information systems (HIS) manage the patient acceptance, personal details, order requests (examination and referral information), scheduling, billing and insurance details, in connection with the hospital administrational information flow. Fluent mutual integration of these information systems should offer more reliable and efficient process flow, and prevent data inconsistencies between systems.
Regarding radiological image review, more and more image interpretation and visualization techniques previously included in the modality based diagnostic workstations are currently handled with PACS workstations. These extended PACS features open better image post-processing and visualization tools for larger number of PACS users, extending from radiologist review to clinician web-based image review. The radiologists are used to look tomographic modality data as multi-planar reformat slices (MPR). However, for clinician or dentist, additional 3D visualizations such as volume rendering, may offer more natural and comprehensive approach into volumetric image data. Modern PACS networks can be adapted for more extensive regional use, where dental practices outside the radiology departments are a good example.
Dentist practices may be connected to a larger regional PACS and RIS systems in order to benefit from the existing radiological IT infrastructure, or it may have systems of its own. Smaller scale image archives with single local workstation and web-based «thin» client access to the image data can be obtained directly from equipment vendors as a reasonably sized investment. However, irrespective of the organisational scale, the maintenance of the PACS and RIS systems should be appropriately performed for information safety and data security, e.g. back-ups, updates, access issues and support. Single dentist practice has rarely professional IT experts of its own. Thus, IT infrastructure and support may be most efficiently acquired as external service.
In Finland, the National Archive of Health Information (project called KanTa) will provide data system services for healthcare providers, pharmacies and all citizens. The services are deployed in phases throughout Finland. In the final phase, the KanTa system includes electronic prescription (currently implemented), pharmaceutical database, health information part for citizens, and patient records archive. Patient record archive will allow data sharing between different users and organizations taking care of strict regulations.
In the future, the coherence of medical IT systems will proceed with more structured and quantitative approach to the «Big Data» that is accumulating in wider scale through basic and specialized healthcare continuum. At the same, time, radiology organizations have to cope with increasing data loads, as diagnostics functions are utilizing increasingly multimodal and 3D/4D imaging methods. This data will need more sophisticated review and analyse tools, extending to automatic computer aided diagnostics (CAD) which will help radiologists to screen relevant findings from the vast amount of diagnostic data. However, the true value of the diagnostic data is measured by the efficacy - how well the radiological examination served the primary indication and clinical question, and how the radiological report contributed in choosing the right treatment to the patient. This is even more essential issue in future, considering the current efforts to improve the cost-effectiveness and pressure for cost cuts throughout the healthcare sector. The increasing need for reliable and more extensive information concerning efficacy and effectiveness will be the fundamental motivators of the future radiological applications and IT solutions.
References
Preston JD. Digital tools for clinical dentistry. An Internet tutorial. J Calif Dent Assoc. 1998 Dec; 26 (12): 915 - 22. Review.
Mol A. Imaging methods in periodontology. Periodontol 2000. 2004; 34: 34 - 48. Review.
Dowsett DJ, Kenny PA, Johnston RE, The Physics of Diagnostic Imaging, 2. edition, Hodder Arnold, UK London, 2006.
Quintero JC, Trosien A, Hatcher D, Kapila S. Craniofacial imaging in orthodontics: historical perspective, current status, and future developments. Angle Orthod. 1999 Dec; 69 (6): 491 - 506.
National Electrical Manufacturers Association (NEMA): http://medical.nema.org/
AAPM. Assessment of Display Performance for Medical Imaging Systems. 2005 edition. AAPM Task Group 18, 2005: 1 - 145.
Butt A, Mahoney M, Savage NW. The impact of computer display performance on the quality of digital radiographs: a review. Aust Dent J. 2012 Mar; 57 Suppl 1: 16 - 23.
Hellen-Halme K, Petersson A, Warfvinge G, Nilsson M. Effect of ambient light and monitor brightness and contrast settings on the detection of approximal caries in digital radiographs. An in vitro study. Swed Dent J. 2007b; Supplement 184.
Abrahams JJ. Dental CT imaging: a look at the jaw. Radiology. 2001 May; 219 (2): 334 - 45.
Vandenberghe B, Jacobs R, Bosmans H. Modern dental imaging: a review of the current technology and clinical applications in dental practice. Eur Radiol. 2010 Nov; 20 (11): 2637 - 55.
Suomalainen A, Kiljunen T, Käser Y, Peltola J, Kortesniemi M. Dosimetry and image quality of four dental cone beam computed tomography scanners compared with multislice computed tomography scanners. Dentomaxillofac Radiol. 2009 Sep; 38 (6): 367 - 78.
Ahmad I. Digital dental photography. Part 1: an overview. Br Dent J. 2009 Apr 25; 206 (8): 403 - 7.
Ahmad I. Digital dental photography. Part 2: Purposes and uses. Br Dent J. 2009 May 9; 206 (9): 459 - 64.
Kauppinen T, Koivikko MP, Ahovuo J. Improvement of report workflow and productivity using speech recognition-a follow-up study. J Digit Imaging. 2008; 21: 378 - 382.
Krishnaraj A, Lee JKT, Laws SA, Crawford TJ: Voice recognition software: Effect on radiology report turnaround time at an academic medical center. Am J Roentgenol. 2010; 195: 94 - 197.
Corresponding author: Mika Kortesniemi, HUS Medical Imaging Center, POB 340, FI-00029 HUS, Finland. E-mail: mika.kortesniemi@hus.fi
Artikkelen har gjennomgått ekstern faglig vurdering. / This article has been peer reviewed.
Kortesniemi M, Ekholm M, Kauppinen T. The handling of digital images. Nor Tannlegeforen Tid. 2014; 124: 120-25.
Artikkelen er fagfellevurdert.
Artikkelen siteres som:
Kortesniemi M, Ekholm M, Kauppinen T, Kortesniemi M, Ekholm M, Kauppinen T. The handling of digital images. Nor Tannlegeforen Tid. 2014;124:120
-25. doi:10.56373/2014-2-10