The stem cell concept in oral mucosa and in cancer
Oral mucosa is a highly dynamic tissue that rapidly replaces its structure and contributes to oral health by maintaining an intact barrier that protects the underlying tissues in the face of much environmental stress. The rapid rate of mucosal renewal has been known for many years but it is only now becoming apparent that mucosal renewal and repair depends ultimately on a small subpopulation of cells, known as stem cells. Only stem cells have the ability to continuously generate new cells for the whole lifetime and when they divide they both renew themselves and produce hierarchies of other cells that differentiate for tissue function. This has many implications for tissue renewal and, of particular interest, important roles of stem cells in the development and treatment of cancer are emerging. Cancers appear to arise as a result of changes in stem cells and altered stem cells are then responsible for driving tumour growth and metastasis. Within a cancer, as in normal tissue, stem cells are few and difficult to identify. This complicates the need to identify their special characteristics and ensure that they are included within the targets of therapeutic procedures. An additional interesting feature of normal stem cells is that they appear to have marked plasticity and, at least under experimental or pathological conditions, they can change to generate many different types of tissues. This is contrary to previous ideas about the stability of cell phenotypes after development, stem cells and offers a range of promising new tissue engineering techniques for the restoration of diseased or damaged tissues.
The oral mucosa is a compound tissue consisting of both epithelial and connective tissue components (1). The stratifying squamous epithelium that forms the surface of the oral mucosa renews its structure throughout life with continuous proliferation of cells in the deeper epithelial layers and produces cells that move upwards as they differentiate to be eventually lost from the surface by desquamation. This process requires a fine balance between the rates of cell formation and cell loss and the mechanisms that control proliferation, differentiation and cell death are of central importance to the maintenance of the normal structure (2). Transient changes that increase cell production can occur during physiological repair and wound healing, and more permanent changes in the normal homeostatic balance underlie a range of neoplastic and other pathological changes (3, 4). Several lines of evidence indicate that the renewal and repair of most, perhaps all, epithelia depends ultimately on a sub-population of the basal cells known as stem cells and that these cells have unusual properties (5 – 8). It is now also apparent that these cells have important roles in the initiation and growth of cancers (4).
The stem cells present in adult tissues, which are known as somatic stem cells, differ in several ways from embryonic stem cells which are cells isolated from the inner cell mass of the blastocyst (9). Embryonic stem cells exist only transiently in vivo but once isolated can be extensively propagated in vitro, are pluripotent, and are able to give rise to almost any type of tissue (10). Somatic stem cells also have the capacity to renew themselves indefinitely but, unlike embryonic stem cells, one of their most important properties is also to produce cells that differentiate for tissue function (11). Maintenance of a constant tissue mass of functional tissue (homeostasis) for the entire lifespan is achieved by the unique pattern of self-renewal of somatic stem cells where each division of a typically produces one cell that remains a stem cell and one cell that enters the differentiation pathway (12,13). How this pattern of «asymmetric» division is controlled is a central problem for stem cell biology (14) and loss of this normal pattern is one of the key changes occurring in malignancy (4). Epithelial stem cells, and those of most other tissues, normally share the properties of extensive self-renewal and generation of proliferative hierarchies of differentiating cells but the number of stem cells and their patterns of distribution seem to vary markedly from one tissue to another (8). Another interesting feature of somatic stem cells is that they act as if they are committed to differentiate into a particular type of tissue. For example, after in vitro culture and re-transplantation back to in vivo sites, stem cells isolated from tissues such as blood, epithelium, cartilage or muscle typically generate only cells with same pattern of differentiation as their tissue of origin (4). This property has been clinically exploited, for example, in the transplantation of bone marrow stem cells to restore haematopoiesis after radiation therapy, in the in vitro growth and grafting of epidermis for burns patients, and in the use of cartilage stem cells for joint regeneration (15,16). However, despite this apparent functional stability, there is now surprising evidence that somatic stem cells are actually quite plastic and may retain multipotent properties (14,17). Under various experimental and pathological conditions, and perhaps sometimes normally, it has been shown that even adult epithelial stem cells are capable of generating a range of different cell lineages (18) and that, conversely, epithelia can be generated from the stem cells of other types of tissue .
General features of stratified squamous epithelia
An epithelium can be characterised by its morphology, its cell turnover, and the cellular products it produces during differentiation. Consequently, as they differentiate, the cells of a renewing epithelium change their histological appearance and their progress towards terminal differentiation can be independently monitored by their changing patterns of expression of various molecules such as cytokeratins. In some epithelial regions the pattern of cell stratification is accompanied by organization of the epithelium into distinct «units of structure». For example, in both human and rodent skin, most regions of the epidermis is organized into stacks of cells that run vertically through the upper strata (19). The epithelia of some regions are organized into larger units of structure, such as tongue papillae and hair, which form distinct anatomical structures with localized regions of cell proliferation (6,20). Even when such complex structures are absent, some form of repeating lateral pattern such as epithelial rete that interdigitate with connective tissue papillae is often characteristic of tissues such as the skin and oral mucosa (3,11).
Epithelial renewal
It was initially suggested that the homeostatic balance between epithelial cell division and cell differentiation was maintained by an essentially random process in which population pressure resulting from cell division caused cells to be randomly squeezed out of the stratum basale to enter the statum spinosum (21). However, the small columnar structures present in mouse epidermis have non-random patterns of cell proliferation suggesting that each of these columnar units functions as an «epidermal proliferative unit» and is actually a clone of cells derived from a single stem cell (19, 22, 23, 24). The basal cell layer thus appears to consist of three functionally-different types of cells: a) a small subpopulation of stem cells, the only basal cells with essentially unlimited self-renewal abilities, b) transit amplifying cells, produced by stem cell divisions and able to undergo a few rounds of cell division before they differentiate, and c) post mitotic differentiated cells preparing to emigrate from the basal layer (3) (Fig. 1). Evidence for the presence of clonal units of epithelial cells arising from individual stem cells has been provided by various studies that have marked the cell lineages arising from individual stem cells (19, 23).
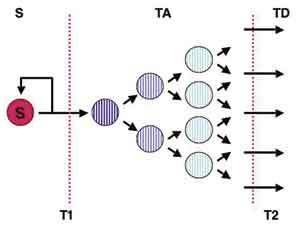
Fig. 1. Illustration of the type of stem cell system hypothesised to function in oral and other epithelia. Three basal cell compartments (stem = S, transient amplifying = TA, and post-mitotic terminally differentiating = TD) are separated by two transitions T1 and T2 (dotted lines). Normally, each stem cell division produces one cell that remains a stem cell (S) and one that becomes a transient amplifying cell and undergoes a series of divisions to produce an expanded number of terminally differentiating cells. The T1 transition controls stem cell homeostasis; for example, lack of transition from S to TA would lead to accumulation of stem cells but increased transition from S to TA would lead to stem cell loss and atrophy of the tissue. The T2 transition controls the number of differentiated cells produced per stem division: a shift of one tier to the left or right halves or doubles, respectively, the total number of cells produced per stem cell division. Typically the direction of cell progression is from left to right but it is uncertain whether reverse transit of cells from TA to S also sometimes occurs normally.
Identification of stem cells
A great deal of effort has been put into trying to find markers that can identify epithelial stem cells but no unique stem cell features have yet been found that readily distinguish them from other basal cells (3,8). The earliest of the various methods developed to identify stem cell distributions was based on the assumption that although epithelial stem cells have the high proliferative potential required for renewal of the epidermis during the entire lifetime of the host organism they would, like blood stem cells, actually divide rarely under normal conditions. As infrequently dividing cells, stem cells would be expected to retain any DNA label such as tritiated thymidine or BrDU that becomes incorporated during division (25). Other cells would also be initially labelled but would either lose their label by dilution as they divide or be lost from the basal population by differentiation. When young mice are injected with tritiated thymidine, most basal cells are initially labelled but only a small population of cells subsequently remained labelled (25,26). These «label-retaining cells» were shown to have the high in vitro proliferative potential anticipated for stem cells and to be able to generate a new epithelium (27). This method has since been used to identify stem cells to the bulge region of hair follicles, to the limbal region of the cornea, to the base of villi in the gut, and to other localized regions in various other tissues (20,28,29). Label retention, although still the only in vivo method to be generally accepted for localizing epithelial stem cells, has two major limitations. Firstly, being dependent on incorporation of a nuclear label, the method cannot be used for studies of human tissues. Secondly, the initial labelling procedure does not label all basal cells and therefore does not subsequently identify all stem cells. However, label-retaining cells in mouse epidermis are found in the centre of the group of 10 – 12 basal cells beneath each columnar units suggesting that there is one stem cell per unit and, consequently, that about 8 – 10 % of basal cells act as stem cells (26,30).
Studies of the distribution of stem cells in human and rodent epithelia often differ in their findings suggesting that the number and pattern of distribution of stem cells differs markedly with species and from one epithelial region to another. In human epidermis stem cells are reported to lie among the basal cells at the tops of the dermal papillae (30) but in oesophagus they are located among the basal cells at the deep tip of the epithelial rete (31). As previously mentioned, the epidermis of rodents consists of small clonal units and label retention indicates that each is regenerated by a single stem cell. Other rodent tissues, however, show different patterns with label retaining cells lying in the «bulge» region of hair follicles and in the ducts of glands (20,28,32). The focal patterns of epithelial stem cell distribution suggest the existence of stem cell «niches», restricted regions where stem cells are able to survive due to interactions with adjacent cells or the matrix (33,34). The «niche» required for haematopoietic stem cell survival requires their contact with osteoblasts but the nature of niches that may support epithelial stem cells is as yet unknown.
Stem cells in oral mucosa
Most of what is assumed about stem cell patterns in oral mucosa has been inferred from work with other epithelia, usually epidermis, but some of the information available has been derived by direct examination. Histologically, human oral epithelia show well-defined patterns of stratification and in some regions are also organized into clearly defined anatomical units of structure. Tongue papillae, for example, are complex structures made up of three distinct regions of differentiation and early kinetic studies of murine mucosa suggested that each of these regions is renewed by stem cells that are positioned at the base of the anterior and posterior columns of the papillae (6,35). That this is the position occupied by stem cells has been confirmed by the presence of label-retaining cells at these sites (25). In other mucosal regions, label-retaining cells are typically located at the deep tips of the epithelial rete ridges, suggesting a stem cell position different from most regions of rodent or human epidermis but similar to palmar epidermis and esophageal epithelium (5,31). The epithelium overlying the rougae of anterior region of human palate has a marked rete pattern with deep and regularly interdigitating projections formed between the epithelium and the connective tissue. The distribution of differentiation markers within the epithelium of this region indicates the presence of three types of cells related to the rete: a) a stem cell zone at the deep tips of the rete, b) a zone of amplifying cells occupying the remainder of the basal layer, and c) the non-proliferative suprabasal cells which express a range of late differentiation markers (Fig. 2). This suggests that stem cells are distributed in relation to the rete but when palatal epithelia are examined by transplantation of reconstructed tissues containing genetically marked stem cells, small clonal units similar to those found in human epidermis (11). However, it is known that complex structures, such as tongue papillae and hair, require information from the sub-adjacent connective tissue for their development and maintenance (36,37) and if experimental reconstruction methods fail to regenerate adequate interactions, normal stem cell patterns may not be re-established. The small clonal units found in experimentally-reconstructed tissues may thus represent the intrinsic pattern of stem cell behaviour from which development of more complex structural patterns is orchestrated by further tissue interactions. The recent demonstration that the epidermis of intact human skin may show only small clonal units unrelated to rete is at variance with the findings of most previous studies and further confuses the issue (24).
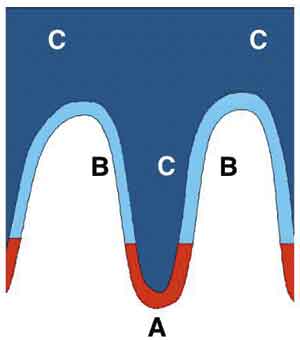
Fig. 2. Pattern of stem cell distribution in oral epithelia. Most regions of keratinizing human oral mucosa has a marked rete pattern and staining for various molecular markers indicates the presence of cells at three different stages of differentiation. Cells in a stem cell zone at the base of the epithelial rete stain for stem cell markers such as keratins 15 and 19. Above this level basal cells stain for early differentiation markers such as keratins 6 and 16. As the cells move suprabasally, they acquire expression of additional differentiation markers such as keratins 1 and 10 (11). The pattern shown here is similar to that proposed for the epithelium lining the oesophagus (31).
Stem cells in tissue engineering and gene therapy
Stem cells are ultimately responsible for all tissue regeneration and clinical techniques that transplant cells for the regeneration of the haematopoietic system, or generate sheets of epidermis in vitro to treat burns patients (16), can be considered, in essence, «stem cell therapies». Typically, however, transplantation therapies have not used purified populations of stem cells but have relied on stem cells being present within the cell population to be transplanted or cultured. Considerable advances have been made in isolating pure populations of haematopoietic stem cells (15) and regeneration of corneal mucosa by isolation and enrichment of limbal stem cells has made sufficient progress to restore sight to severely damaged eyes (38). As stem cells have a high regenerative potential, tissue regenerated from isolated stem cells is expected to have better survival properties (39). The development of successful and durable gene therapy also depends on the successful isolation and manipulation of stem cells to maintain transgene expression and the feasibility of using epithelial stem cells for somatic gene therapy has been demonstrated (40). The feasibility of in vitro expansion of populations of oral epithelial cells prior to transplantation back to the oral cavity to heal oral wounds has been shown but, as yet, therapies based specifically on oral stem cells have not yet been developed (41, 42).
Under some experimental circumstances somatic stem cells have been shown to possess extensive plasticity. For example, when injected into developing mouse embryos, even stem cells from adult epithelia are able to re-differentiate and participate in the formation of many organs, suggesting that these cells may possess some of the plasticity of embryonic stem cells (18). This has raised further interest in their use for tissue engineering but there are suggestions that some of the changes seen in somatic stem cells may result from cell fusion rather than re-differentiation (43,44). The degree of functional plasticity of somatic stem cells therefore remains uncertain and controversy persists concerning the relative values of somatic and embryonic stem cells for tissue engineering (17). Clinically however, plasticity of haematopoietic stem cell is apparent and female patients who have received transplants of male bone marrow have been shown to generate buccal epithelial cells containing the male X-chromosome (45).
The possibility of generating new tissues from embryonic and somatic stem cells has raised practical interest in the processes underlying the development of tissues and organs. Concerning oral tissues in particular, the molecular mechanisms associated with the development of teeth are now quite well understood and are being related to the possibility of generating new teeth for clinical use (46, 47). Recent reports indicate that combining developmental information with principles of tissue engineering may enable the differentiation of adult stem cells into functional dental structures (48, 49).
Stem cells in oral tumours
Currently there is great interest in the roles of stem cells in cancer with recent studies indicating that stem cells act both as the functional target of carcinogens and, after malignant change, as the source of cells driving the continuing growth of cancers (4,50). Stem cell hierarchies have been found in leukaemias where only a small fraction of leukaemic cells represents «tumour initiating cells» that have the ability to regenerate the tumour (15). Similar malignant stem cell patterns are present in breast cancers (51) and hierarchical stem cell patterns can even be demonstrated in cell lines derived from other tumours (52, 53). Stem cell hierarchies can also be demonstrated in cell lines derived from oral cancers and the persistence of essentially normal stem and amplifying colony forms indicate the extremely basic nature of this pattern (53). The stem and amplifying cells from a range of tissues have markedly differing patterns of motility, apoptosis, drug exclusion and gene expression and marked differences also persist between the stem and amplifying cells of cell lines (53). The importance of these observations lies in their implications for therapy; if malignant stem cells are the driving force of tumour growth then they are the cells that need to be eradicated but at present there are few methods for monitoring the responses of malignant stem cells to therapy (4,51, 53).
References
1. Schroeder HE. Differentiation of human oral stratified epithelia. Basel: Karger S; 1981.
2. Hume WJ. Stem cells in oral epithelia. Stem cells: Their identification and characterization. Potten CS, editor. Edinburgh: Churchill Livingstone; 1983. p. 233 – 70.
3. Watt FM. Epidermal stem cells: markers, patterning and the control of stem cell fate. Philos Trans R Soc Lond B Biol Sci 1998; 353: 831 – 7.
4. Reya T, Morrison SJ, Clarke MF, Weissman IL. Stem cells, cancer, and cancer stem cells. Nature 2001; 414: 105 – 11.
5. Lavker RM, Sun TT. Heterogeneity in epidermal basal keratinocytes: morphological and functional correlations. Science 1982; 215: 1239 – 41.
6. Hume WJ, Potten CS. Proliferative units in stratified squamous epithelium. Clin Exp Dermatol 1983; 8: 95 – 106.
7. Hall PA, Watt FM. Stem cells: the generation and maintenance of cellular diversity. Development 1989; 106: 619 – 33.
8. Cotsarelis G, Kaur P, Dhouailly D, Hengge U, Bickenbach J. Epithelial stem cells in the skin: definition, markers, localization and functions. Exp Dermatol 1999; 8: 80 – 8.
9. Evans M, Hunter S. Source and nature of embryonic stem cells. C R Biol 2002; 325: 1003 – 7.
10. Niwa H. Molecular mechanism to maintain stem cell renewal of ES cells. Cell Struct Funct 2001; 26: 137 – 48.
11. Tudor D, Locke M, Owen-Jones E, Mackenzie IC. Intrinsic patterns of behavior of epithelial stem cells. J Invest Dermatol 2004; 9: 208 – 14.
12. Lajtha G. Stem cell concepts. Differentiation 1979; 14: 23 – 34.
13. Potten CS, Morris RJ. Epithelial stem cells in vivo. J Cell Sci 1988; 10 (Suppl): 45 – 62.
14. Pardal R, Clarke MF, Morrison SJ. Applying the principles of stem-cell biology to cancer. Nat Rev Cancer 2003; 3: 895 – 902.
15. Dick JE. Stem cells: Self-renewal writ in blood. Nature 2003; 423: 231 – 3.
16. Compton CC. Cultured human sole-derived keratinocyte grafts re-express site-specific differentiation after transplantation. Differentiation 1998; 64: 45 – 53.
17. Prockop DJ. Further proof of the plasticity of adult stem cells and their role in tissue repair. J Cell Biol 2003; 160: 807 – 9.
18. Liang L, Bickenbach JR. Somatic epidermal stem cells can produce multiple cell lineages during development. Stem Cells 2002; 20: 21 – 31.
19. Mackenzie IC. Retroviral transduction of murine epidermal stem cells demonstrates clonal units of epidermal structure. J Invest Dermatol 1997; 109: 377 – 83.
20. Cotsarelis G, Cheng SZ, Dong G, Sun TT, Lavker RM. Existence of slow-cycling limbal epithelial basal cells that can be preferentially stimulated to proliferate: implications on epithelial stem cells. Cell 1989; 57: 201 – 9.
21. Potten CS. The epidermal proliferative unit: the possible role of the central basal cell. Cell Tissue Kinet 1974; 7: 77 – 88.
22. Potten CS. Cell replacement in epidermis (keratopoiesis) via discrete units of proliferation. Int Rev Cytol 1981; 69: 271 – 318.
23. Ghazizadeh S, Taichman LB. Organization of stem cells and their progeny in human epidermis. J Invest Dermatol 2005; 124: 367 – 72.
24. Bickenbach JR. Identification and behavior of label-retaining cells in oral mucosa. J Dent Res 1981; 60: 1611 – 20.
25. Bickenbach JR, McCutecheon J, Mackenzie IC. Rate of loss of tritiated thymidine label in basal cells in mouse epithelial tissues. Cell Tissue Kinet 1986; 19: 325 – 33.
26. Bickenbach JR, Chism E. Selection and extended growth of murine epidermal stem cells in culture. Exp Cell Res 1998; 244: 184 – 95.
27. Cotsarelis G, Sun TT, Lavker RM. Label-retaining cells reside in the bulge area of pilosebaceous unit: implications for follicular stem cells, hair cycle, and skin carcinogenesis. Cell 1990; 61: 1329 – 37.
28. Potten CS, Loeffler M. Stem cells: attributes, cycles, spirals, pitfalls and uncertainties. Lessons for and from the crypt. Development 1990; 110: 1001 – 20.
29. Morris RJ, Potten CS. Slowly cycling (label-retaining) epidermal cells behave likeclonogenic stem cells in vitro. Cell Prolif 1994; 27: 279 – 89.
30. Jensen UB, Lowell S, Watt FM. The spatial relationship between stem cells and their progeny in the basal layer of human epidermis: a new view based on whole-mount labelling and lineage analysis. Development 1999; 126: 2409 – 18.
31. Seery JP, Watt FM. Asymmetric stem-cell divisions define the architecture of human oesophageal epithelium. Curr Biol 2000; 10: 1447 – 50.
32. Rochat A, Kobayashi K, Barrandon Y. Location of stem cells of human hair follicles by clonal analysis. Cell 1994; 76: 1063 – 73.
33. Watt FM. Stem cell fate and patterning in mammalian epidermis. Curr Opin Genet Dev 2001; 11: 410 – 7.
34. Spradling A, Drummond-Barbosa D, Kai T. Stem cells find their niche. Nature 2001; 414: 98 – 104.
35. Hume WJ, Potten CS. Changes in proliferative activity as cells move along undulating basement membranes in stratified squamous epithelium. Br J Dermatol 1980; 103: 499 – 504.
36. Mackenzie IC. Epithelial-mesenchymal interactions in the development and maintenance of epithelial tissue. In: Lane EB, Leigh I, Watt FM, editors. The keratinocyte handbook. Cambridge: Cambridge University Press; 1994.
37. Cunha GR, Bigsby RM, Cooke PS, Sugimura Y. Stromal-epithelial interactions in adult organs. Cell Differ 1985; 17: 137 – 48.
38. Di Iorio E, Barbaro V, Ruzza A, Ponzin D, Pellegrini G, De LM. Isoforms of {Delta}Np63 and the migration of ocular limbal cells in human corneal regeneration. Proc Natl Acad Sci USA 2005; 102: 9523 – 8.
39. Hengge UR, Taichman LB, Kaur P, Rogers G, Jensen TG, Goldsmith LA, et al. How realistic is cutaneous gene therapy? Exp Dermatol 1999; 8: 419 – 31.
40. Ghazizadeh S, Taichman LB. Virus-mediated gene transfer for cutaneous gene therapy. Hum Gene Ther 2000; 11: 2247 – 51.
41. De Luca M, Albanese E, Megna M, Cancedda R, Mangiante PE, Cadoni A. Evidence that human oral epithelium reconstituted in vitro and transplanted onto patients with defects in the oral mucosa retains properties of the original donor site. Transplantation 1990; 50: 454 – 9.
42. Langdon J, Williams DM, Navsaria H, Leigh IM. Autologous keratinocyte grafting: a new technique for intra-oral reconstruction. Br Dent J 1991; 171: 87 – 90.
43. Terada N, Hamazaki T, Oka M, Hoki M, Mastalerz DM, Nakano Y, et al. Bone marrow cells adopt the phenotype of other cells by spontaneous cell fusion. Nature 2002; 416: 542 – 5.
44. Ying QL. Changing potency by spontaneous fusion. Nature 2002; 416: 545 – 8.
45. Tran SD, Pillemer SR, Dutra A, Barrett AJ, Brownstein MJ, Key S, et al. Differentiation of human bone marrow-derived cells into buccal epithelial cells in vivo: a molecular analytical study. Lancet 2003; 361: 1084 – 8.
46. Thesleff I, Tummers M. Stem cells and tissue engineering: prospects for regenerating tissues in dental practice. Med Princ Pract 2003; 12 Suppl 1: 43 – 50.
47. Tummers M, Thesleff I. Root or crown: a developmental choice orchestrated by the differential regulation of the epithelial stem cell niche in the tooth of two rodent species. Development 2003; 130: 1049 – 57.
48. Ohazama A, Modino SA, Miletich I, Sharpe PT. Stem-cell-based tissue engineering of murine teeth. J Dent Res 2004; 83: 518 – 22.
49. Modino SA, Sharpe PT. Tissue engineering of teeth using adult stem cells. Arch Oral Biol 2005; 50: 255 – 8.
50. Morris RJ. Keratinocyte stem cells: targets for cutaneous carcinogens. J Clin Invest 2000; 106: 3 – 8.
51. Al-Hajj M, Wicha MS, ito-Hernandez A, Morrison SJ, Clarke MF. Prospective identification of tumorigenic breast cancer cells. Proc Natl Acad Sci USA 2003; 100: 3983 – 8.
52. Mackenzie IC. Growth of malignant oral epithelial stem cells after seeding into organotypical cultures of normal mucosa. J Oral Pathol Med 2004; 33: 71 – 8.
53. Locke M, Heywood M, Fawell S, Mackenzie IC. Retention of intrinsic stem cell hierarchies in carcinoma-derived cell lines. Cancer Res 2005; 65: 8944 – 50.
Correspondance: Professor Sally Dabelsteen, Department of Oral Medicine, Clinical Oral Physiology, Oral Pathology & Anatomy, School of Dentistry, 20 Noerre Allé, DK-2100 Copenhagen, Denmark. E-mail: sally@odont.ku.dk
Artikkelen har gjennomgått ekstern faglig vurdering.