How to build a tooth? Developmental biology is revealing the instructions
Forfatter
DDS. Dr. odont. Director of the Developmental Biology Research Program, Institute of Biotechnology, University of Helsinki, Finland
Artikkelen har tidligere stått publisert i: Schou (ed). Nordic Dentistry 2003 Yearbook. Copenhagen: Quintessence; 2003: 117 – 28.
Developmental biology is at present one of the most rapidly progressing fields in biology and biomedicine. The advances of gene technology have led to a rapid explosion in the understanding of the molecular mechanisms regulating embryonic development. New genes and their functions are continuously being discovered in experimental studies using animal embryos, and molecular genetic studies in humans are unravelling gene mutations causing congenital defects. Today animal models can be created for human diseases and new possibilities are opening up for prevention, diagnosis and treatment of congenital defects. In addition there is now great hope that the knowledge on the molecules driving tissue and organ development and cell differentiation will lead to tools for tissue regeneration and stem cell therapies in the future. It may even be possible to grow whole new organs such as teeth.
I entered the exciting field of developmental biology 30 years ago as an undergraduate dental student and have been fortunate to be part of its dramatic progress, which really can be called a revolution. I received excellent training from my thesis supervisor Lauri Saxén in experimental embryology. In the 1980´s it became possible to study development at the level of genes, and with excellent students in my group we combined gene technology with classical methods of experimental embryology. We dissected dental tissues from mouse embryos and cultured them in various conditions, and studied the expression of genes. Over the years we have analysed the functions of many different genes by various approaches. Recently we have used transgenic mouse technology to examine the functions of some genes, in particular genes which affect the number of teeth in humans (see below).
The fascinating concept of a common molecular tool-kit regulating development evolved during the 1980´s and 1990´s. It was realized that the same genes regulate developmental processes in all animals and in all different organs and tissues. Our group contributed to this concept by unraveling the roles that many important molecules belonging to the conserved developmental tool-kit have in teeth (Jernvall and Thesleff, 2000, Thesleff, 2000, Thesleff and Mikkola, 2002). This indicated that the teeth are no exception of the rule; on the contrary, it is noteworthy that no «tooth-specific» regulatory molecules have so far been identified (besides some which are involved in the formation of dentin and enamel). In other words, all currently known genes that affect the position, shape or number of teeth have developmental regulatory functions also in other tissues.
Signaling molecules: Key regulators of tooth development
The special aim of our research has been to elucidate the mechanisms whereby cells communicate during development. It has been known for more than a century that cells signal to each other and thereby direct cells to new pathways and thereby controlled the advancement of development. This has been called embryonic induction and today this is believed to be the single most important mechanism of developmental regulation. Many embryologists showed already in the 1950´s and 1960´s that intercellular signaling is an important feature of tooth development, and that in teeth the signaling events take place mainly between the epithelial and mesenchymal tissue components (Fig 1, Kollar and Baird, 1969, Ruch et al., 1983, Thesleff and Nieminen, 2001).
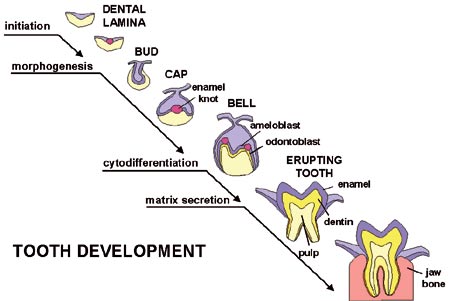
Fig 1. The morphogenesis of a tooth. Interactions between the epithelial and mesenchymal tissues regulate advancing development.
The developmental signal molecules are small proteins which usually act by binding on specific receptors at the surface of the responding cell (Fig 2). A multistep intracellular cascade leads to regulation of gene expression in the nucleus and the cell then changes its behaviour. Our work has pinpointed the roles of several signal molecules and their targets during the early steps of tooth development when teeth are inititiated and their shapes are determined. We have proposed a model on how conserved signaling molecules mediate sequential and reciprocal interactions between dental epithelium and mesenchyme and thereby regulate advancing tooth morphogenesis (Fig 3). The model is based on results from many laboratories including our own. The most studied signals belong to four different families including fibroblast growth factor (FGF), bone morphogenetic proteins (BMP), hedgehog and WNT. In addition to signals, the model in Fig 3 also includes several genes which are regulated by the signals in the responding tissues. Mutations in many of these genes have already been shown to cause dental defects in mice as well as in humans (see below).
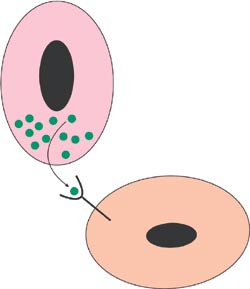
Fig 2. Secreted signal molecules are the most important mediators of cell communication during development. Binding of the signal to a specific receptor at the surface of the responding cell leads to changes in its behavior.
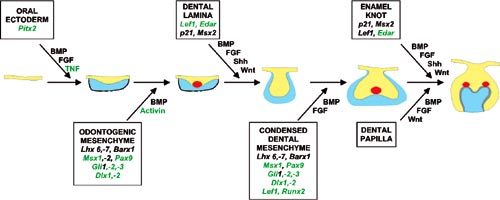
Fig 3. Model of the molecular regulation of tooth development from initiation to crown morphogenesis. Interactions between epithelial (blue) and mesenchymal tissues (yellow) are mediated by signal molecules (BMP, bone morphogenetic proteins, FGF, fibroblast growth factors, SHH, sonic hedgehog, WNT, TNF, tumor necrosis factor). These signals operate throughout development and regulate the expression of genes in the responding tissues (shown in the boxes). Signaling centers (red) appear in the epithelium reiteratively and secrete locally more than ten different signals which regulate morphogenesis and tooth shape. The genes in green color have been shown to be necessary for normal tooth development.
A breakthrough in our research was the discovery that BMP is an important signal during the initiation of tooth development. BMP is synthesized by the early dental epithelium, and it induces in the mesenchyme the expression of many genes including the transcription factor Msx1 (Vainio et al., 1993). For this study an organ culture method was designed in which the local effects of signal molecules are analysed by introducing them with small beads on developing dental tissues (Fig 4). Tooth germs were dissected from mouse embryos and epithelial and mesenchymal tissues were separated and placed on metal grids covered with culture medium. The beads were incubated in a high concentration of BMP protein and placed on dental mesenchyme and the explant was cultured for one day in an incubator. Thereafter the expression of candidate target genes was examined by in situ hybridization from whole mounts (Fig 5). Later it was shown by others that Msx1 is, in fact, a necessary gene for tooth development both in mice and humans. No teeth developed in so called knockout mice lacking the function of Msx1. Their tooth development was arrested at the bud stage (Satokata and Maas, 1994). In humans mutations in the MSX1 gene cause severe oligodontia (eight or more missing teeth) (Vastardis et al., 1996).
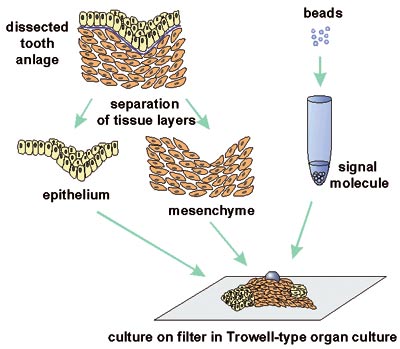
Fig 4. The in vitro method developed for the analysis of local effects of signal molecules (See text for the description).
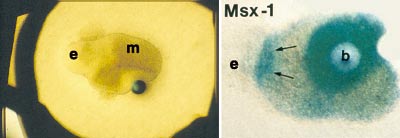
Fig 5. Effects of BMP signals on dental tissues cultured as shown in Fig 4 A. Light microscopic view of an explant of combined epithelium (e) and mesenchyme (m) and a bead releasing BMP signal. B. Induction of Msx1 expression around the bead and in the mesenchyme adjacent to epithelium. Whole mount in situ hybridization analysis of a similar explant as in Fig. 4 A.
Enamel knots: Signalling centers governing tooth shape
An important leap forward in our studies was the discovery of signalling centers, called enamel knots in the tooth germ epithelium (Jernvall et al., 1994). Although the enamel knots had been described already a century earlier in morphological studies as clusters of epithelial cells in the cap stage tooth organs, their function was not known. The first signal discovered in the enamel knot was FGF-4, and today more than ten different signals belonging to the four families mentioned above have been localized in enamel knots (Fig 2 and 6). Jukka Jernvall has continued the studies on enamel knots and has shown that the enamel knots instruct the patterning of the tooth crowns. They determine the location and height of tooth cusps by inducing new, secondary enamel knots at the sites of future cusps. His recent results indicate that slight changes in enamel knot signaling can explain the differences between the shapes of molars in various mammalian species and that such changes can also account for the evolutionary changes in dental morphology that are well known from the fossil record (Jernvall et al., 2000, Salazar and Jernvall, 2002). In Fig 6 B the expression of p21 gene in the secondary enamel knots prefigures the formation of cusps in a mouse molar.
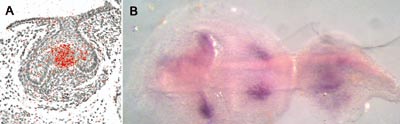
Fig 6 A. The primary enamel knot is visualized in a frontal section through the cap stage tooth germ (expression of Edar, the receptor for ectodysplasin). B. Secondary enamel knots of the bell stage first molar (left) prefigure cusps. The second molar (right) is at cap stage and the primary enamel knot is seen. (p21 expression, occlusal view of whole mount in situ hybridization).
Gene expression in teeth: A database at www.bite-it.helsinki.fi
During the years we and others have reported the expression patterns of numerous genes during tooth development. Most data were obtained by in situ hybridization, a method detecting the sites of mRNA expression in tissues. As the information of gene epression patterns was accumulating with increasing speed, Pekka Nieminen in our group constructed in 1995 an Internet database to help ourselves as well as the whole scientific community to keep track on genes expressed in teeth (Nieminen et al., 1998, www.bite-it.helsinki.fi). This graphical database shows gene expression patterns at various stages of tooth development and in addition it contains supplementary data on important genes. At present the database includes information of about 350 genes. As an example, the page on Msx1 expression is shown in Fig 7. The database is now widely used internationally and members of our laboratory keep it updated.
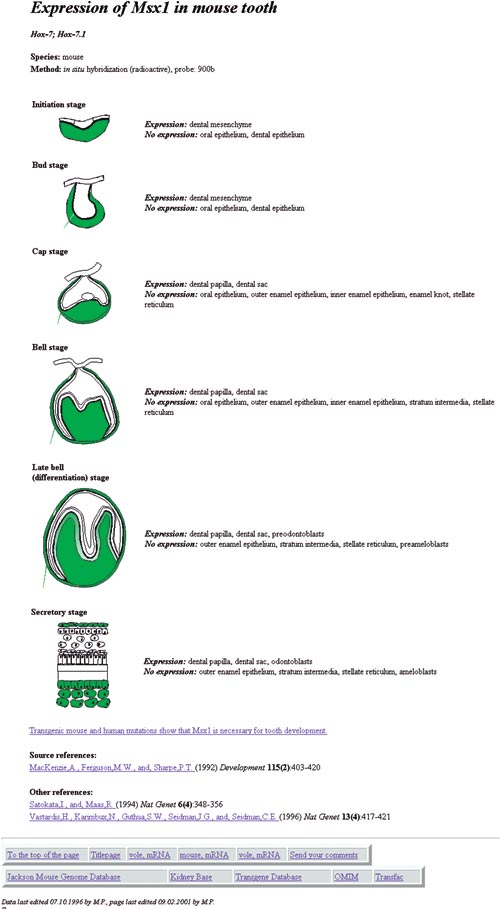
Fig 7. The page describing the expression pattern of the Msx1 gene in the Internet database «Gene expression in teeth» at www.bite-it.helsinki.fi
Although the information on the patterns of gene expression do not directly tell about gene function, they give important insights. For example, the co-expression of several signal molecule genes in the enamel knots actually led to the unraveling of the function of the enamel knot as a signaling center of teeth. Today, our gene expression database is widely used as a tool to detect co-expression of genes and to discover molecular interactions between genes.
The cleidocranial dysplasia gene RUNX2: A promise for the induction of new teeth
Cleidocranial dysplasia is a syndrome affecting bone and tooth development and it is caused by mutations in RUNX2 (earlier called CBFA1), a gene encoding a transcription factor. The symptoms include hypoplastic bone, in particular deficient formation of calvarial bones and clavicles. The tooth phenotype is particularly interesting as the patients have supernumerary teeth, and sometimes an almost complete third dentition develops (Jensen et al.,1990). This indicates, remarkably, that all of us have the potential to develop a third dentition and that this is normally inhibited by the RUNX2 gene.
RUNX2 is a «master gene» of bone development and it is needed for osteoblast differentiation. RUNX2 knockout mice have no bone at all and their skeleton is composed of only cartilage. We studied the tooth phenotype of these mice and quite unexpectedly observed that their teeth failed to develop (Fig 8). Teeth were initiated but their development was arrested after the bud stage indicating that RUNX2 function is necessary for bud to cap stage transition (D´Souza et al., 1999).
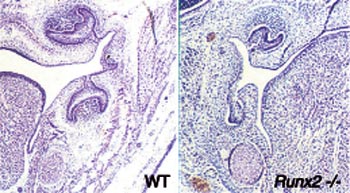
Fig 8. The Runx2 gene is necessary for tooth development. The deletion of its function in knockout mice (Runx2 -/-) arrests tooth development at the bud stage. In normal mice (wild type, WT) at the same age tooth germs have reached the cap stage.
Cleidocranial dysplasia is caused by reduced function of RUNX2. That supernumerary teeth develop in these patients whereas the complete loss of RUNX2 function in mice inhibits tooth formation appears controversial, but it is a challenging problem. Unfortunately, the mice do not normally develop a secondary dentition at all and therefore they cannot be used as model animals for studies on secondary tooth formation. However, we hope that by clarifying the function of RUNX2 in primary tooth development in mice we can shed light to the mechanisms whereby the secondary dentition develops in humans, and that this will also explain why the third dentition develops in the human cleidocranial dysplasia patients.
We are now analysing RUNX2 knockout mice and aim to clarify the cause of arrested tooth development. We are searching for genes which are regulated by RUNX2 by comparing gene expression between the mutants and wildtype mice. We use microarray technology and DNA chips which allows the simultaneous analysis of thousands of genes including also presently unknown genes. Could this information be used for inducing a new set of teeth in the future?
Ectodysplasin, the molecule lacking in ectodermal dysplasia: A stimulator of tooth formation
Ectodermal dysplasia syndromes are characterized by absence or hypoplastic development of several ectodermal organs. In addition to teeth these organs include hairs, nails and a variety of exocrine glands such as sweat glands, salivary glands and mammary glands. The development of all these organs are regulated by epithelial mesenchymal interactions which are mediated by the same signals as tooth development (Pispa and Thesleff, 2003). The most common form is the hypohidrotic or anhidrotic ectodermal dysplasia (HED). The tooth phenotype of these patients includes severe hypodontia, sometimes complete anodontia (Fig 8). Several gene mutations causing HED have been identified recently by positional cloning of the human and corresponding mouse mutants (Thesleff and Mikkola, 2002). Interestingly these genes function in the tumor necrosis factor (TNF) signal pathway. X-chromosomal HED is caused by mutations in the actual TNF signal, called ectodysplasin, and other forms result from mutations in the ectodysplasin receptor (Edar) and other components in the same signal pathway.
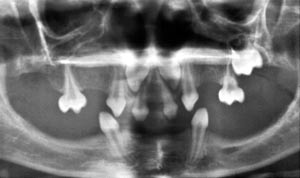
Fig 9. Oligodontia in a patient with hypohidrotic (anhidrotic) ectodermal dysplasia (HED).
We have shown that ectodysplasin regulates the functions of the enamel knots and hair placodes (which are signaling centers for hair formation) (Pispa et al., 1999, Laurikkala et al., 2000, 2001). We have also used several mouse mutants in these studies including the natural occurring ectodysplasin knockout called the Tabby mouse. In addition we have produced transgenic mice overexpressing either ectodysplasin or its receptor Edar in the ectoderm. Interestingly, while the third molars are mostly missing in the Tabby mice, the ectodysplasin overexpressing mice have extra teeth (Fig 10, Mustonen et al., 2003). Hence, ectodysplasin is an important signal regulating tooth number and shape. Since ectodysplasin is a secreted protein it is tempting to imagine that it could be used in the future to induce new teeth and hairs in ectodermal dysplasia patients. Could it be used to grow new teeth in other patients too?
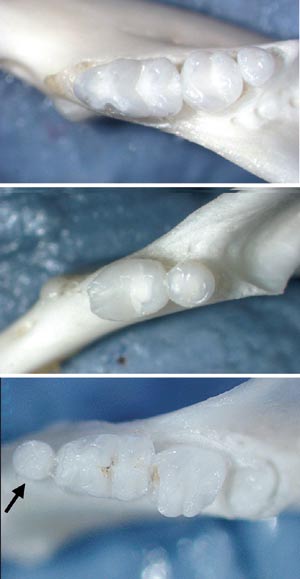
Fig 10. Ectodysplasin stimulates tooth formation. A. Normal mice have three molars. B. Tabby mouse mutants which lack ectodysplasin gene function lack the third molar. C. Mice with increased function of ectodysplasin gene have an extra tooth in front of the first molar.
Prospects for growing new teeth: Dental stem cells and signal molecules
A frequent question asked after my lectures and seminars over the years has been: «When can you grow new teeth for us?». Earlier I used to say «never», but more recently, due to the fantastic progress in the fields of developmental biology and stem cell biology, I have started to be more optimistic. This is first of all due to the rapid increase in our understanding of the molecular regulation of tooth development. Of particular importance here is the identification of the signal molecules such as ectodysplasin and many others guiding tooth development at different stages (Fig 3). Secondly, the recent scientific breakthroughs in stem cell research have indicated that adult cells may be much more plastic in their behavior than previously thought (Donovan and Gearhart, 2001, Jiang et al., 2002). In other words, cells may change the directions of differentiation according to environmental signals. The interest in tissue regeneration in general has increased tremendously and data is accumulating rapidly on the competence of various cell types to be programmed to different fates and on the signals which induce their differentiation.
Stem cells have been identified also in teeth. Mesenchymal stem cells which formed dentin when transplanted to muscle were found in the adult dental pulp (Gronthos et al., 2001). These cells could perhaps provide the mesenchymal tissue for bioengineering teeth. We have identified epithelial stem cells in the cervical loop of the continuously growing mouse incisors and shown that their maintenance and differentiation depends on FGF signals (Fig 11, Harada et al., 1999). Unfortunately, the human teeth do not grow continuously and they do not contain a pool of differentiating epithelial cells that could be used for growing new teeth. However, the characterization of the stem cells in mice may lead to the discovery of suitable human cells that could be triggered to take part in tooth development.
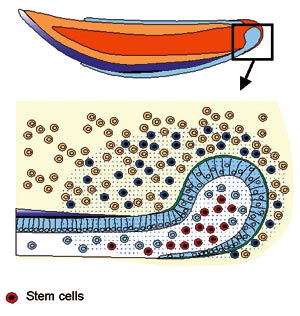
Fig 11. Stem cells are present in the cervical loop of the continuously growing mouse incisor. Their maintenance and differentiation depends on FGF-10 signal secreted by the dental mesenchyme.
It is obvious that it will take a long time before teeth can be grown in dental practice. It will still require more knowledge on the molecules regulating tooth development. This is the specific area where we are continuing research in Helsinki. Also, more research is needed in order to find ways to identify and harvest potential stem cells and to induce their differentiation to specific directions. In addition, the practical designs for transplantation and culture of cells and tissues present great challenges. However, the dreams about tooth regeneration may after all come true some day in the future.
Dyrking av kunstige tenner: Science eller fiction?
Få, om noen områder kan vise til en slik utvikling som bioteknologien har vært igjennom de siste årene. Metoder som for kort tid siden ble ansett som «science fiction», har i høyeste grad kvittet seg med «fiction»-begrepet, og molekylærbiologiske teknikker ser nå ut til å åpne for totalt nye muligheter innen både diagnostikk og behandling av sykdommer. Utviklingsbiologiens oppgave i denne sammenhengen er bl.a. å beskrive de prosesser og signalveier som fører til at ulike vev og organer dannes. Arbeidet som er gjort innen dette feltet har identifisert flere mekanismer som er nødvendige for vellykket og synkronisert utvikling av et organ eller sogar et individ. Det har også ført til identifisering av flere enkeltgener som, når de er uttrykt på feil sted eller til feil tid, kan føre til store misdannelser. Kartleggingen av disse mekanismene kan på sikt gi oss mulighet til å dyrke frem «kunstige» organer. Dette vil naturligvis kunne ha stor betydning ved agenesier eller patologiske prosesser. Tannanlegget er en klassisk og svært velegnet modell for å beskrive signalveiene som fører til dannelsen av et funksjonelt organ. Den systematiske forskningen som er utført innen dette feltet gjør også tennene til aktuelle kandidater for organer som lar seg fremstille kunstig. For som artikkelen antyder: Kjenner man mekanismene bak den naturlige utviklingen, har man også oppskriften.
Professor Irma Thesleff har i en årrekke figurert blant verdens ledende utviklingsbiologer, med særlig fokus på mekanismer som regulerer tannutviklingen. Hun ble tildelt Anders Jahres store medisinske pris for 1999.
References
Donovan PJ, Gearhart J. The end of the beginning for pluripotent stem cells. Nature 2001; 414: 92 – 97.
D´Souza RN, Åberg T, Gaikwad J, Cavender A, Owen M, Karsenty G, Thesleff I. Cbfa 1 is required for epithelial-mesenchymal interactions regulating tooth development in mice. Development 1999; 126: 2911 – 2920.
Gronthos S, Mankani M, Brahim J, Robey PG, Shi S. Postnatal human dental pulp stem cells (DPSCs) in vitro and in vivo. Proc Nat Acad Sci USA 2000; 97: 13625 – 13630.
Harada H, Kettunen, P, Jung HS, Mustonen T, Wang YA, Thesleff I. Localization of putative stem cells in dental epithelium and their association with Notch and FGF signaling. J Cell Biol 1999; 147: 105 – 120.
Jensen BL, Kreiborg S. Development of the dentition in cleidocranial dysplasia. J Oral Pathol Med 1990; 19: 89 – 93.
Jernvall J, Keränen SV, Thesleff I. Evolutionary modification of development in mammalian teeth: Quantifying gene expression patterns and topography. Proc Nat Acad Sci USA 2000; 97: 14444 – 14448.
Jernvall J, Kettunen P, Karavanova I, Martin LB, Thesleff I. Evidence for the role of the enamel knot as a control center in mammalian tooth cusp formation: Non-dividing cells express growth stimulating Fgf-4 gene. Int J Dev Biol 1994; 38: 463 – 469.
Jernvall J, Thesleff I. Reiterative signaling and patterning during mammalian tooth morphogenesis. Mech Dev 2000; 92: 19 – 29.
Jiang Y, Jahagirdar BN, Reinhardt RL, Schwartz RE, Keene CD, Ortiz-Gonzales XR, et al. Pluripotency of mesenchymal stem cells derived from adult marrow. Nature 2002; 418: 41 – 49.
Kollar EJ, Baird GR. The influence of the dental papilla on the development of tooth shape in embryonic mouse tooth germs. J Embryol Exp Morphol 1969; 21: 131 – 148.
Laurikkala J, Mikkola M, Mustonen T, Åberg T, Koppinen P, Pispa J, Nieminen P, Galceran J, Grosschedl R, Thesleff I. TNF signaling via the ligand-receptor pair ectodysplasin and edar controls the function of epithelial signaling centers and is regulated by Wnt and activin during tooth organogenesis. Dev Biol 2001; 229: 443 – 455.
Laurikkala J, Pispa J, Jung HS, Nieminen P, Mikkola M, Wang X, Saarialho-Kere U, Galceran J, Grosschedl R, Thesleff I. Development and disease. Regulation of hair follicle development by the TNF signal ectodysplasin and its receptor Edar. Development 2002; 129: 2541 – 2553.
Mustonen T, Pummila M, Kangas A, Mikkola M, Pispa J, Pakkasjärvi L, Pispa J, Jaatinen R, Thesleff I. Ectodysplasin-A1 stimulates the development of ectodermal organs (submitted). Dev Biol 2003; 253: 123 – 136.
Pispa J, Jung HS, Jernvall J, Kettunen P, Mustonen T, Tabata MJ, Kere J, Thesleff I. Cusp patterning defect in Tabby mouse teeth and its partial rescue by FGF. Dev Biol 1999; 216: 521 – 534.
Pispa J. Thesleff I. Mechanisms of ectodermal organogenesis. Dev Biol 2003; 262: 195 – 205.
Nieminen P, Pekkanen M, Åberg T, Thesleff I. A graphical www-database on gene expression in tooth. Eur J Oral Sci 1998; 106: 7 – 11.
Ruch JV, Lesot H, Karcher-Djuriric V, Meyer JM, Mark M. Epithelial-mesenchymal interactions in tooth germs: mechanisms of differentiation. J Biol Buccale 1983; 11: 173 – 183.
Salazar-Ciudad I, Jernvall J. A gene network model accounting for development and evolution of mammalian teeth. Proc Nat Acad Sci USA 2002; 99: 8116 – 8120.
Satokata I, Maas R. Msx1 deficient mice exhibit cleft palate and abnormalities of craniofacial and tooth development. Nat Genet 1994; 6: 348 – 356.
Thesleff I. Genetic basis of tooth developmental and dental defects. Acta Odont Scand 2000; 58: 191 – 194.
Thesleff I, Mikkola M. The role of growth factors in tooth development. Int Rev Cytol 2002; 217: 93 – 135.
Thesleff I, Nieminen P. Tooth induction. In: Encyclopedia of Life Sciences. 2001; www.els.net.Nature Publishing Group, Macmillan Publisher Ltd. Electronic citation.
Vainio S, Karavanova I, Jowett A, Thesleff I. Identification of BMP-4 signal mediating secondary induction between epithelial and mesenchymal tissue during early tooth development. Cell 1993; 75: 45 – 58.
Vastardis H, Karimbux N, Guthua SW, Seidman JG, Seidman CE. A human MSX1 homeodomain missense mutation causes selective tooth agenesis. Nat Genet 1996; 13: 417 – 421.
Søkeord for nettversjon: www.tannlegetidende.no: Biologi; Emalje; Dentin; Forskning; Genteknologi; Molekylærbiologi
Address: Institute of Biotechnology, POB 56, University of Helsinki, 00014 Helsinki, Finland